Abstract
The paper describes results from an experimental and theoretical study of the effect of an electric field on nucleate boiling and the critical heat flux (CHF) in pool boiling of R123 at atmospheric pressure on a horizontal wall with a smooth surface. Two designs of electrode (parallel rods and wire mesh) were used. The experimental data exhibit some differences from the data obtained by other researchers in similar experiments on a wall with a different surface finish and with a slightly different design of wire mesh electrode. The hydrodynamic model for EHD enhancement of CHF cannot reconcile the differences. A theoretical model has been developed for the growth of a single vapour bubble on a superheated wall in an electric field, leading to a numerical simulation based on the level-set method. The model includes matching of sub-models for the micro- and macro-regions, conduction in the wall, distortion of the electric field by the bubble, the temperature dependence of electrical properties and free-charge generation. In the present form of the model, some of these effects are realised in an approximate form. The capability to investigate dry-spot formation and wall temperature changes that might lead to CHF has been demonstrated.












Similar content being viewed by others
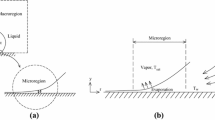
Abbreviations
- \(\bar{A}\) :
-
Hamaker constant, J
- A :
-
empirical constant
- B :
-
empirical constant
- c P :
-
specific heat at constant pressure (J/(kg K))
- d :
-
characteristic length (m)
- E :
-
electric field strength (V/m)
- El :
-
electric influence number
- E l′:
-
electric influence number
- El * :
-
electric influence number
- f l :
-
fugacity
- g :
-
gravitational vector (m/s2)
- H :
-
Heaviside step function
- h :
-
grid spacing (m)
- h 0 :
-
heat transfer coefficient without electric field
- h E :
-
heat transfer coefficient with electric field
- \(h_{{ev}} = 2{\sqrt {\frac{M}{{2\pi \bar{R}T_{v}}}}}\frac{{\rho_{v} h^{2}_{{fg}}}}{{T_{v}}}\) :
-
evaporation heat transfer coefficient (W/(m2 K))
- h fg :
-
latent heat of evaporation (J/kg)
- HV :
-
applied high voltage (kV)
- k :
-
thermal conductivity (W/(m K))
- M :
-
molecular weight (kg/mol)
- m :
-
mass flux vector (kg/(m2 s))
- \(\dot{m}_{{\rm mic}} \) :
-
evaporation rate from the microlayer (kg/s)
- Nu :
-
Nusselt number
- Pr :
-
Prandtl number
- p :
-
pressure (N/m2)
- \(\bar{p}\) :
-
empirical constant
- Q :
-
integrated heat flux (W/m)
- q :
-
heat flux (W/m2)
- q cr (E) = q E :
-
critical heat flux with electric field (kW/m2)
- q cr (0) = q 0 :
-
critical heat flux without electric field, (kW/m2)
- r :
-
radial coordinate (m)
- r c :
-
half-width of zone in (48) (m)
- R :
-
radius of computational domain (m)
- \(\bar{R}\) :
-
universal gas constant
- R o :
-
radius of dry region beneath a bubble (m)
- R 1 :
-
radial location of the interface at y = h/2 (m)
- t :
-
time (s)
- T :
-
temperature (K)
- ΔT = T w − T sat :
-
wall superheat (K)
- u :
-
velocity vector (u,v)
- u :
-
r-directional velocity (m/s)
- v :
-
y-directional velocity (m/s)
- V :
-
bubble volume (m3)
- V c :
-
control volume (m3)
- V l :
-
molar volume (m3/mol)
- y :
-
vertical coordinate (m)
- Y :
-
height of computational domain (m)
- α K :
-
coefficient of thermal increase of electric conductivity R123 (1/K)
- β T :
-
coefficient of thermal expansion (1/K)
- Γ:
-
mass flow rate in the microlayer (kg/s)
- δ:
-
thickness (m)
- δ0 :
-
initial thickness (m)
- δɛ (ϕ):
-
delta function
- δ T :
-
thermal boundary layer thickness (m)
- ɛ:
-
electric permittivity (F/m)
- ɛ0 :
-
electric permittivity vacuum (F/m)
- ɛ r :
-
relative electric permittivity
- κ:
-
interface curvature (1/m)
- λ d :
-
wavelength (m)
- μ:
-
dynamic viscosity (N s/m2)
- ρ:
-
mass density (kg/m3)
- ρ e :
-
electric charge density (C/m3)
- σ:
-
surface tension (N/m)
- τ:
-
charge relaxation time (s)
- σ e :
-
electric conductivity (S)
- ϕ:
-
level set function
- Φ:
-
electric potential (V)
- φ:
-
apparent contact angle (deg)
- ψ:
-
initial bubble shape profile
- int:
-
interface
- l:
-
liquid
- sat:
-
saturation
- v:
-
vapour
- w:
-
wall interface
References
Zuber N (1958) On the stability of boiling heat transfer. Trans ASME J Heat Transfer 90:711–720
Haramura Y, Katto Y (1983) A new hydrodynamic model of critical heat flux, applicable widely to both pool and forced convection boiling on submerged bodies in saturated liquids. Int J Heat Mass Transfer 26:389–399
Nishio S, Gotoh N, Nagai N (1998) Observation of boiling structures in high heat-flux boiling. Int J Heat Mass Transfer 41:3191–3201
Chung HJ, No HC (2003) Simultaneous visualization of dry spots and bubbles for pool boiling of R-113 on a horizontal heater. Int J Heat Mass Transfer 46:2239–2251
Theofanous TG, Tu JP, Dinh AT, Dinh TN (2002) The boiling crisis phenomenon Part I: nucleation and nucleate boiling heat transfer. Exp Thermal Fluid Sci 26:755–792
Theofanous TG, Dinh TN, Tu JP, Dinh AT (2002) The boiling crisis phenomenon Part II: dryout dynamics and burnout. Exp Thermal Fluid Sci 26:793–810
Sefiane K, Benielli D, Steinchen A (1998) A new mechanism for pool boiling crisis, recoil instability and contact angle influence. Colloids Surfaces A Physicochem Eng Aspects 142:361–373
Sefiane K (2001) A new approach in the modeling of the critical heat flux and enhancement techniques. AIChE J 47:2402–2412
Nikolayev VS, Beysens DA (1999) Boiling crisis and non-equilibrium drying transition. Europhys Lett 47:345–351
Nikolayev VS, Chatain D, Beysens D (2006) Is CHF triggered by the vapor recoil effect?, 2006 ECI Conf. on Boiling Heat Transfer, Spoleto, Paper 136, 2006. Update reference to paper in special issue
Wayner PC Jr, Kao YK, Lacroix LV (1976) The interline heat transfer coefficient of an evaporating wetting film. Int J Heat Mass Transfer 19:487–492
Stephan P, Hammer J (1994) A new model for nucleate boiling heat transfer. Heat Mass Transfer 30:119–125
Son G, Dhir VK, Ramanujapu N (1999) Dynamics and heat transfer associated with a single bubble during nucleate boiling on a horizontal surface. Trans ASME J Heat Transfer 121:623–631
Mukherjee A, Dhir VK (2004) Study of lateral merger of vapor bubbles during nucleate pool boiling. Trans ASME J Heat Transfer 126:1023–1039
Mathieu B, Lebaigue O, Tadrist L (2002) Numerical investigation of a dynamic contact line model for perfectly wetting liquids on a heated wall of finite conductivity. Proc 12th Int Heat Transfer Conf Grenoble 3:455–460
Mathieu B, Lebaigue O, Tadrist L, Influence of a dynamic contact line model on the characteristics of nucleate wall boiling computed with a DNS approach. In: Proceedings of 5th international conference on multiphase flow, Yokohama, Paper 439
Allen PHG, Karayiannis TG (1995) Electrohydro-dynamic enhancement of heat transfer and fluid flow—review paper. Heat Recovery Syst CHP 15(5):389–423
Di Marco P, Grassi W (2006) Effect of force fields on pool boiling flow patterns, 2006 ECI Conf. on Boiling Heat Transfer, Spoleto, Paper 136, 2006. Update reference to paper in special issue
Ohadi MM, Sharaf N, Nelson DA (1991) Electrohydrodynamic enhancement of heat transfer in a shell-and-tube heat exchanger. Exp Heat Transfer 14:19–39
Ogata J, Yabe A (1993) Augmentation of boiling heat transfer by utilizing the EHD effect–EHD behaviour of boiling bubbles and heat transfer characteristics. Int J Heat Transfer Mass 36:783–791
Markels M, Durfee RL (1964) The effect of applied voltage on boiling heat transfer. AIChE J 10:106–110
Landau LD, Lifshitz EM (1960) Electrodynamics of continuous media. Addison-Wesley, Reading
Zaghdoudi MC, Lallemand M (2000) Study of the behaviour of a bubble in an electric field: steady shape and local fluid motion. Int J Thermal Sci 39:39–52
Zaghdoudi MC, Lallemand M (2002) Electric field effects on pool boiling. J Enhanced Heat Transfer 9:187–208
Oh S-D, Kwak H-Y (2000) A study of bubble behaviour and boiling heat transfer enhancement under electric field. Heat Transfer Eng 21:33–45
3M Product information sheet
Xu Y (1995) Effects of electric fields on pool boiling heat transfer. PhD Thesis, South Bank University, UK
Karayiannis TG (1998) EHD boiling heat transfer enhancement of R123 and R11 on a tube bundle. Appl Thermal Eng 18:809–817
Karayiannis TG, Xu Y (1998) Electric field effect in boiling heat transfer. Part A: simulation of the electric field and electric forces. J Enhanced Heat Transfer 5:217–229
Karayiannis TG, Xu Y (1998) Electric field effect in boiling heat transfer. Part B: Electrode geometry. J Enhanced Heat Transfer 5:231–247
Uemura M, Nishio S, Tanasawa I (1990) Enhancement of pool boiling heat transfer by static electric field. In: Proceedings of 9th international heat transfer conference, Jerusalem, vol 4, pp 75–80
Ahsmann G, Kronig R (1950) The influence of electric fields on the convective heat transfer in liquids. Appl Sci Res A 2:235–244
Bonjour E, Verdier J, Weil L (1962) Electro-convection effects on heat transfer. Chem Eng Progress 58:63–66
Johnson RL (1968) Effect of an electric field on boiling heat transfer. AIAA J 6:1456–1460
Berghmans J (1976) Electrostatic fields and the maximum heat flux. Int J Heat Mass Trans 19:791–797
Lovenguth RF, Hanesian D (1971) Boiling heat transfer in the presence of nonuniform, direct current electric fields. Ind Eng Chem Fundamentals 10:570–576
Iacona E, Herman C, Chang S, Liu Z (2006) Electric field effect on bubble detachment in reduced gravity environment. Exp Thermal Fluid Sci. doi:10.1016/j.expthermflusci.2006.03.029.
Liu Z, Herman C, Mewes D (2006) Visualization of bubble detachment and coalescence under the influence of a nonuniform electric field. Exp Thermal Fluid Sci. doi:10.1016/j.expthermflusci.2006.03.025
Herman C, Iacona E (2004) Modeling of bubble detachment in reduced gravity under the influence of electric fields and experimental verification. Heat Mass Transfer 40:943–957
Cheng KJ, Chaddock JB (1986) Maximum size of bubbles during nucleate boiling in an electric field. Int J Heat Fluid Flow 7:278–282
Kumar R, Kuloor NR (1970) The formation of bubbles and drops. Adv Chem Eng 8:256–368
Cho HJ, Chang IS, Kweon YC, Kim MH (1996) Study of the behaviour of a bubble attached to a wall in a uniform electric field. Int J Multiphase Flow 22:909–922
Di Marco P, Grassi W, Memoli G, Takamasa T, Tomiyama A, Hosokawa S (2003) Influence of electric field on single gas-bubble growth and detachment in microgravity. Int J Multiphase Flow 29:559–578
Auracher H, Marquardt W (2004) Heat transfer characteristics and mechanisms along entire boiling curves under steady state and transient conditions. Int J Heat Fluid Flow 25:223–242
Yamashita K, Yabe A (1997) Electrodynamic enhancement of falling film evaporation heat transfer and its long-term effect on heat exchangers. Trans ASME J Heat Transfer 119:339–347
Israelachvili J (1992) Intermolecular forces, 2nd edn. Academic, Washington
Gorla RSR, Gatica JE, Ghorashi B, Ineure P, Byrd L (2004) Heat transfer in a thin liquid film in the presence of an electric field. Chem Eng Comm 191:718–731
Sefiane K, Hristov Y, Kenning DBR, Karayiannis TG, Zhao D (2006) Modelling of the growth and detachment of a vapour bubble and the effect of an electric field in nucleate boiling regime. In: 13th International Heat Transfer Conference, 13–18 August, Sydney
Hristov Y, Zhao D, Sefiane K, Karayiannis TG, Kenning DBR (2005) Critical heat flux (CHF) modelling in pool boiling heat transfer, including the effect of an electric field. In: Proceedings of 9th UK National Conference of Heat Transfer, Manchester
Acknowledgments
This work was supported by the Engineering and Physical Sciences Research Council Grants GRS41814 and GR. R37129.
Author information
Authors and Affiliations
Corresponding author
Rights and permissions
About this article
Cite this article
Hristov, Y., Zhao, D., Kenning, D.B.R. et al. A study of nucleate boiling and critical heat flux with EHD enhancement. Heat Mass Transfer 45, 999–1017 (2009). https://doi.org/10.1007/s00231-007-0286-z
Received:
Accepted:
Published:
Issue Date:
DOI: https://doi.org/10.1007/s00231-007-0286-z