Abstract
Ultra-smooth nanocrystalline diamond (UNCD) films with high-acoustic wave velocity were introduced into ZnO-based surface acoustic wave (SAW) devices to enhance their microfluidic efficiency by reducing the acoustic energy dissipation into the silicon substrate and improving the acoustic properties of the SAW devices. Microfluidic efficiency of the ZnO-based SAW devices with and without UNCD inter layers was investigated and compared. Results showed that the pumping velocities increase with the input power and those of the ZnO/UNCD/Si devices are much larger than those of the ZnO/Si devices at the same power. The jetting efficiency of the droplet was improved by introducing the UNCD interlayer into the ZnO/Si SAW device. Improvement in the microfluidic efficiency is mainly attributed to the diamond layer, which restrains the acoustic wave to propagate in the top layer rather than dissipating into the substrate.











Similar content being viewed by others
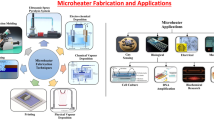
References
Anna SL, Mayer HC (2006) Microscale tipstreaming in a microfluidic flow focusing device. Phys Fluids 18:121512
Bonn D, Eggers J, Indekeu J et al (2009) Wetting and spreading. Rev Mod Phys 81(2):739–805
Brunet P, Baudion M, Bou Matar O et al (2010) Droplet displacements and oscillations induced by ultrasonic surface acoustic waves: a quantitative study. Phys Rev E 81:036315
Campbell CK (1998) Surface acoustic wave devices for mobile and wireless communications. Academic Press, New York
Doinikov AA (1996) Theory of acoustic radiation pressure for actual fluids. Phys Rev E 54(6):6297–6303
Du XY, Fu YQ, Tan SC et al (2008) ZnO film thickness effect on surface acoustic wave modes and acoustic streaming. Appl Phys Lett 93:094105
Du XY, Fu YQ, Luo JK et al (2009a) Microfluidic pumps employing surface acoustic waves generated in ZnO thin films. J Appl Phys 105:024508
Du XY, Swanwick ME, Fu YQ et al (2009b) Surface acoustic wave induced streaming and pumping in 128° Y-cut LiNbO3 for microfluidic applications. J Micromech Microeng 19:035016
Eggers J, Villermaux E (2008) Physics of liquid jets. Rep Prog Phys 71:03660
Flewitt AJ, Dutson JD, Beecher P et al (2009) Stability of thin film transistors incorporating a zinc oxide or indium zinc oxide channel deposited by a high rate sputtering process. Semicond Sci Technol 24(8):085002
Franke TA, Wixforth A (2008) Microfluidics for miniaturized laboratories on a chip. Chem Phys Chem 9:2140–2156
Franke T, Abate AR, Weitza DA et al (2009) Surface acoustic wave (SAW) directed droplet flow in microfluidics for PDMS devices. Lab Chip 9:2625–2627
Friend JR, Yeo LY (2011) Microscale acoustofluidics: microfluidics driven via acoustics and ultrasonics. Rev Mod Phys 83(2):647–704
Fu YQ, Luo JK, Du XY et al (2010) Recent developments on ZnO films for acoustic wave based bio-sensing and microfluidic applications: a review. Sens Actuators B 143:606–619
Fu YQ, Garcia-Gancedo L, Pang HF et al (2012) Microfluidics based on ZnO/nanocrystalline diamond surface acoustic wave devices. Biomicrofluidics 6:024105
García-Gancedo L, Pedrós J, Zhu Z et al (2012) Room-temperature remote-plasma sputtering of c-axis oriented zinc oxide thin films. J Appl Phys 112:014907
Glass NR, Shilton RJ, Chan PPY et al (2012) Miniaturized Lab-on-a-Disc (miniLOAD). Small 8:1881–1888
Guttenberg Z, Müller H, Habermüller H et al (2005) Planar chip device for PCR and hybridization with surface acoustic wave pump. Lab Chip 5:308–317
Hashimoto K (2011) Demands of highly piezoelectric materials for radio frequency acoustic wave devices. Phys Stat Sol (a) 208(5):1084–1092
Jopp J, Grull H, Yerushalmi-Rozen R (2004) Wetting behavior of water droplets on hydrophobic microtextures of comparable size. Langmuir 20(23):10015–10019
Kestin J, Sokolov M, Wakeham WA (1978) Viscosity of liquid water in the range −8–150 °C. J Phys Chem Ref Data 7(3):941–948
Le Brizoual L, Sarry F, Moreira F et al (2006) FEM modelling of surface acoustic wave in diamond layered structure. Phys Stat Sol (a) 203(12):3179–3184
Luo JK, Fu YQ, Le HR et al (2007) Diamond and diamond-like carbon MEMS. J Micromech Microeng 17:S147–S163
Marcelli R, Rossi M, De Gasperis P (1993) End reflections and triple transit contribution to the band shape of magnetostatic volume wave delay lines. J Appl Phys 73:3082–3086
Mark D, Haeberle S, Roth G et al (2010) Microfluidic lab-on-a-chip platforms: requirements, characteristics and applications. Chem Soc Rev 39:1153–1182
McMurdie H, Morris M, Evans E et al (1986) Standard X-Ray diffraction powder patterns from the JCPDS Research Associateship. Powder Diffr 1(2):76–77
Mitsuyu T, Ono S, Wasa K (1980) Structures and SAW properties of rf-sputtered single-crystal films of ZnO on sapphire. J Appl Phys 51:2464–2470
Mortet V, Williams OA, Haenen K (2008) Diamond: a material for acoustic devices. Phys Stat Sol (a) 205(5):1009–1020
Mulligan MK, Rothstein JP (2011) The effect of confinement-induced shear on drop deformation and breakup in microfluidic extensional flows. Phys Fluids 23:022004
Nakahata H, Fujii S, Higaki K et al (2003) Diamond-based surface acoustic wave devices. Semicond Sci Technol 18:S96–S104
Nakahata H, Hachigo A, Higaki K et al (2005) Effect of crystalline quality of diamond film to the propagation loss of surface acoustic wave devices. IEEE Trans Ultrason Ferroelectr Freq Control 52(10):1817–1822
Nguyen N-T, Wu Z (2005) Micromixers—a review. J Micromech Microeng 15:R1–R16
Olsson RH III, El-Kady I (2009) Microfabricated phononic crystal devices and applications. Meas Sci Technol 20:012002
Pang HF, Garcia-Gancedo L, Fu YQ, Porro S, Gu YW, Luo JK, Zu XT, Placido F, Wilson JIB, Flewitt AJ, Milne WI Surface acoustic wave devices based on ZnO/ultra-nanocrystalline diamond structures. Phys Stat Sol (a) (submitted)
Pedrós J, García-Gancedo L, Ford CJB et al (2011) Guided propagation of surface acoustic waves and piezoelectric field enhancement in ZnO/GaAs systems. J Appl Phys 110:103501
Porro S, De Temmerman G, Lisgo S et al (2009) Nanocrystalline diamond coating of fusion plasma facing components. Diam Relat Mater 18(5–8):740–744
Qi A, Yeo LY, Friend JR (2008) Interfacial destabilization and atomization driven by surface acoustic waves. Phys Fluids 20:074103
Rayleigh L (1879) On the capillary phenomena of jets. Proc Roy Soc Lond 29:71–97
Reboud J, Bourquin Y, Wilson R et al (2012) Shaping acoustic fields as a toolset for microfluidic manipulations in diagnostic technologies. PNAS 109(38):15162–15167
Renaudin A, Tabourier P, Zhang V et al (2006) SAW nanopump for handling droplets in view of biological applications. Sens Actuators B 113(1):389–397
Shi J, Huang H, Stratton Z et al (2009) Continuous particle separation in a microfluidic channel via standing surface acoustic waves (SSAW). Lab Chip 9:3354–3359
Smith WR, Gerard HM, Collins JH et al (1969) Analysis of interdigital surface wave transducers by use of equivalent circuit model. IEEE Trans Microwave Theory Tech MTT-17(11):856–864
Squires TM, Quake SR (2005) Microfluidics: fluid physics at the nanoliter scale. Rev Mod Phys 77(3):977–1026
Tan MK, Friend JR, Yeo LY (2007) Microparticle collection and concentration via a miniature surface acoustic wave device. Lab Chip 7(5):618–625
Tan MK, Friend JR, Yeo LY (2009) Interfacial jetting phenomena induced by focused surface vibrations. Phys Rev Lett 103:024501
Tang I-T, Wang YC, Hwang WC et al (2003) Investigation of piezoelectric ZnO film deposited on diamond like carbon coated onto Si substrate under different sputtering conditions. J Cryst Growth 252(1–3):190–198
Tsai P, Lammertink RGH, Wessling M et al (2010) Evaporation-triggered wetting transition for water droplets upon hydrophobic microstructures. Phys Rev Lett 104(11):116102
Vargaftik NB, Volkov BN, Voikak LD (1983) International table of the surface tension of water. J Phys Chem Ref Data 12(3):817–820
Wixforth A, Strobl C, Gauer C et al (2004) Acoustic manipulation of small droplets. Anal Bioanal Chem 379:982–991
Wood CD, Cunningham JE, O’Rorke R et al (2009) Formation and manipulation of two-dimensional arrays of micron-scale particles in microfluidic systems by surface acoustic waves. Appl Phys Lett 94:054101
Yeo L, Friend JR (2009) Ultrafast microfluidics using surface acoustic waves. Biomicrofluidics 3(1):012002
Yoon SH, Huang Y, Edgar JS et al (2012) Surface acoustic wave nebulization facilitating lipid mass spectrometric analysis. Anal Chem 84:6530–6537
Acknowledgments
The authors acknowledge support from the Fundamental Research Funds for the Central Universities (ZYGX2009J046 and ZYGX2009X007), and the Sichuan Young Scientists Foundation (2010JQ0006), the Royal Society-Research Grant (RG090609), Carnegie Trust Funding, Royal Society of Edinburgh, Royal Academy of Engineering-Research Exchanges with China and India Awards. L. Garcia-Gancedo, A.J. Flewitt and W.I. Milne acknowledge the financial support of the EPSRC, through grants number EP/F063865/1 and EP/F06294X/1. L. García-Gancedo also acknowledges the support from the National Natural Science Foundation of China (NSFC) through grant number 61150110485.
Author information
Authors and Affiliations
Corresponding authors
Rights and permissions
About this article
Cite this article
Pang, HF., Fu, Y.Q., Garcia-Gancedo, L. et al. Enhancement of microfluidic efficiency with nanocrystalline diamond interlayer in the ZnO-based surface acoustic wave device. Microfluid Nanofluid 15, 377–386 (2013). https://doi.org/10.1007/s10404-013-1155-3
Received:
Accepted:
Published:
Issue Date:
DOI: https://doi.org/10.1007/s10404-013-1155-3