Introduction
A series of deep drill holes was sunk in the spring of 1956 in Salmon Glacier, in the Coast Mountains of British Columbia near the south-eastern tip of Alaska (long. 130° 10′ W., lat. 56° 09′ N.). Drilling was done by Granduc Mines Limited, a company engaged in the exploration of a large copper deposit in a nunatak 40 km. north-west of the seaport town of Stewart, B.C. Tunnelling between Stewart and the mine has been considered as one possible means of providing access. To ensure that the tunnel would pass beneath Salmon Glacier the company decided, after an unsuccessful attempt at a seismic survey, to drill holes in the ice to establish the bedrock profile along the tunnel line. As easy access to the drilling site was limited to winter and spring, at which time water for rotary drills would not readily be available, it was decided to undertake thermal drilling. After preliminary studies in the fall and winter of 1955–56, equipment was prepared and hauled by tractor to the glacier. Drilling continued till June 1956. The first hole was abandoned at a depth of 323 m. without reaching the bottom of the glacier, but 5 other holes were sunk (Fig. 1) to depths of from 495 m. to 756 m. before obstacles were reached that could not be penetrated after 24 hours drilling. These obstacles were assumed to be the bedrock floor of the glacier, although it was recognized that large boulders embedded within the ice might similarly prevent penetration of the hot point. Later successful seismic studies by a University of Toronto geophysical team indicated that these obstacles were indeed either the bedrock surface or morainal debris very close to it.

Fig. 1. Plan and section of Salmon Glacier, British Columbia, in vicinity of proposed Granduc tunnel line. Map after ont prepared by the photogrammetry section, National Research Council, Ottawa, Ont. (contour interval 10 m.)
Drilling was conducted by means of a Calrod thermal element sheathed in copper, 3 in. (7.6 cm.) in diameter, powered by a 220 volt, 10 kW. Onon portable generator with a variable voltage transformer. The hot point was suspended from the surface by means of a 3/16 in. (4.7 mm.) wire rope to which the electric cable was clamped. In drilling through firn the element would be operated at reduced voltage, but after the hot point entered ice the power input would be increased to 220 volts and 8 amp. which permitted an average penetration of close to 1.8 m. per hour (see Fig. 2). Operations were conducted through the floor of an insulated plywood hut which also served as living quarters for the drill crew of 2 men. Apart from brief halts for maintenance or for replacement of burnt-out elements, drilling continued 24 hours a day until a hole was completed. Each hour the hot point would be lowered to the bottom of the hole and 10 ft. (3.05 m.) of slack cable paid off; at the end of the next hour the remaining slack would be withdrawn, depth of penetration noted, and 10 ft. of slack again paid out. The first hole, drilled by this procedure became so crooked that it had to be abandoned, and in later work a 20 foot (6.1 m.) length of pipe was rigidly attached behind the heating element to guide it in its descent. This device greatly reduced crookedness but the survey of the 4th hole (Fig. 2) indicated that once the drill unit was deflected from the vertical it tended to continue off plumb. Difficulties were encountered in drill hole No. 3 at about 460 m. because of rock fragments, one of which was retrieved by fishing, but in all holes other than this one and the first the drilling continued with no significant problems until the bottom was reached.
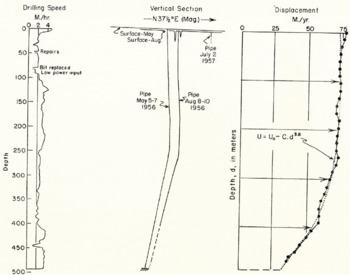
Fig. 2. Drilling speeds, vertical section, and rate of movement with depth at Salmon Glacier No. 4 drill hale
Advised of the proposed drilling in the fall of 1955, the writer made a request to the Associate Committee on Soil and Snow Mechanics of the National Research Council, Ottawa, Ontario, for financial aid in casing and surveying one of the holes. This request was quickly granted. Granduc Mines agreed to cooperate in transporting and installing the casing. Accordingly 2,000 ft. (610 m.) of

Surveys
Notified of the successful installation of the casing, the writer was flown to Salmon Glacier and completed the first survey on 4–7 May. The collar of the hole was located relative to three fixed points on the valley walls (black triangles in Fig. 1) by means of a Wild T-1 transit. The pipe was surveyed at 50 ft. (15.25 m.) intervals with a Parsons inclinometer (SharpReference Sharp 1 ) which records photographically at a set time the position of a compass and pendulum suspended within the instrument. With a long pendulum in this inclinometer a drift (deviation from the vertical) of up to 4° can be measured with an accuracy of about 05′ of arc; with a shorter pendulum a drift of up to 10° can be measured with an accuracy of about 10’ of arc. In all cases the azimuth can be read to about 5°. Unfortunately the pipe deviated more than 10° from the vertical below the 259 m. level and only the azimuth of the drift could be measured by means of the Parsons inclinometer in this first survey. Thanks, however, to good radio communication, equipment was flown to the site by Granduc Mines to complete a survey of the lower part of this hole by means of acid bottles.
A second visit was made to the site on 7–11 August 1956. On this occasion crevasses made aircraft landings near the drill site impossible and in any event poor weather had suspended flying. Accordingly we carried equipment and instruments to the drill hole from the end of the nearest road, 16 km. away. Survey stations on the valley walls were reoccupied and the inclinometer survey repeated. An extra short pendulum, which permitted observations of drift of as much as 27° with an accuracy of 30′ of arc was used below the 247 m. level. After the survey was completed to the 396 m. level the inclinometer was damaged, and in the absence of spare parts only incomplete readings of drift without azimuth could be obtained, and these at 30.5 m. intervals, down to the lowest point by now accessible, 446.5 m. from the surface. In computing positions of points on the pipe the assumption was made that the movement since May below 396 m. was in the same direction,

Plans were made during the winter of 1956–57 to add more anti-freeze to the pipe and, if necessary, to add an extra 20 foot length of pipe, but by the time the first tractor trains were operating on Salmon Glacier in January a timber tripod 8 m. high standing over the collar of the pipe was completely buried.
A third visit was made between 30 June and 4 July 1957, with transit, inclinometer, and thermistor gear for a temperature survey. The timber tripod was found without difficulty although it had been severely crushed by winter snow and only the uppermost half meter was protruding. After considerable digging the pipe was located beneath the remains of the timber mat. The pipe, when opened, proved to be plugged, apparently by ice, at a depth of to 10.4 m., and as no equipment could be obtained to thaw the obstructions, surveys within the hole had to be abandoned. The top of the pipe was again located relative to the survey stations on the valley walls and steps taken to measure the longitudinal surface velocity gradient.
Observations and Conclusions
The site of the cased drill hole is on a relatively flat and regular stretch of Salmon Glacier, about 610 m. from the south side and 1,830 m. from the north side (Fig. 1). Surface elevation here was 1,315 m. above sea-level (May 1956) and surface slope very close to 2° 00′. Oblique crevasses are common; one 2 m. south-west of the pipe extended to an estimated depth of 25 m. Judging both from air photos taken in September 1950, and from the shallow depth of firn existing in August 1956, the site lies very close to the firn line. The head of the glacier lies 5 km. to the south-west, the more distant of the two termini lies 11 km. to the south-east. The valley walls have an average slope of close to 30° and rise 600 to 1000 m. above the ice. Drilling has indicated that the glacier reaches a depth of roughly 730 m., and this figure has since been confirmed by seismic studies.
Surface movement of the drill hole amounted to 21.2 m. in 94 days between May and August 1956 (0.225 m./day) and to 88.5 m. in 423 days between May 1956 and July 1957 (0.209 m./day). Observations on a line of stakes at the drill site between 3 and 31 July 1957, indicated a longitudinal extension averaging 7 per cent per year. Observed surface displacements at drill holes 2 and 5 over the 94 day period in 1956 amounted to 25.9 m. and 23.5 m. respectively (or 0.275 m. /day and 0.250 m./day) only a little more than at the cased hole. Surface displacement at Hole 4 between May and August 1956, thus seems reasonably representative both of the long term movement and of this cross section of the glacier. Direction of flow during the first 94 day period was



Positions at 16.4 m. (50.0 ft.) intervals down the pipe have been computed for both the 1956 surveys, assuming smooth curvature of the pipe between successive points of known inclination. The amount and direction of displacement of each of these points were then determined. Small adjustments have been made to convert from slope distance to vertical distance. Further correction has also proved necessary to allow for the original inclination of the pipe for as NyeReference Nye 2 points out: “If the pipe were initially normal to the surface it would begin to tilt in proportion to the shear-strain rate … but, once tilted, the longitudinal extension rate would produce a further tilt on its own account.” The data have thus been adjusted and plotted (Fig. 2) to show what would have been the deflections of a straight pipe originally normal to the ice surface. As shown the maximum velocity was found to occur at surface, but at mid depth the velocity was only 8 per cent less than at surface. At the lowest level attainable, 13 m. from the bottom of the hole and

Numerous minor irregularities in the depth-velocity curve are noted, but most of these may have no significance. Many of the irregularities are no larger than the possible errors of measurement of inclination, and the assumption of a smoothly curving pipe between points of known attitude need not hold. However, the inflection above the 30 m. depth may have resulted from local and incipient collapse of the serac in which the hole was drilled.
The problem of ice flowing past the pipe at a rate greater (or, locally, less) than that of the pipe itself has been discussed by Gerrard et al. Reference Gerrard, Perutz and Roch 3 It can only be added that any layer of ice of significant thickness moving faster, or slower, than adjacent layers would produce a bend in the pipe which, if large enough to be detected, would reveal the existence if not the velocity of the layer in question. The present study thus offers no encouragement to Demorest’s hypothesis Reference Demorest 4 , Reference Demorest 5 of “extrusion flow” at depth.
The depth-velocity curve obtained for Salmon Glacier corresponds rather closely (Fig. 2) to the theoretical curve for laminar flow (NyeReference Nye 6 p. 83–84):

in which u 0 is the velocity of the surface layer, u the velocity at depth d, and α the angle of surface slope. For the Salmon Glacier data the best fit is obtained with u 0=78 m./yr. rather than 82 m./yr., the observed surface velocity, additional evidence that this surface velocity may have been influenced by incipient collapse of the serac into which the hole was drilled. For the same data, n proves to be close to 2.8 and using the surface slope of 2° 00′, K proves to be about 1.25×10−4. The standard deviation of the displacements from a smooth curve corresponding to this formula is, except for the uppermost 61 m. of the hole, 1.3 m./yr. This close correspondence between observed displacements and the curve based on Nye’s formulaReference Nye 6 does not, of course, prove that the flow is laminar, but it does at least show that the formula gives a close approximation of real behaviour even at great depth within a valley glacier. To what extent the coefficient K and the exponent n are influenced by environment, notably temperature, will become apparent with additional field and laboratory studies elsewhere.
Extrapolation of the velocity data, using Nye’s formula, to the bottom of the glacier at 495 m. indicates a basal velocity of 34 m./yr., or 44 per cent of u 0.
The shear stress τ parallel to the glacier surface has been computed according to the formula:

using the same density distribution as was accepted by GerrardReference Gerrard, Perutz and Roch 3 down to the 140 m. depth and a constant density of 0.92 gm./cm.3 below that. The maximum value of this shear stress, at the bottom of the glacier, is 1.42 bars, higher than averageReference Nye 7 but within the accepted limits.
No temperature records were obtained from the hole and conditions, particularly at depth, can be inferred only by comparison. On the Taku Glacier, in a similar environment 350 km. to the north-west, 1,090 m. above sea-level, MillerReference Miller 8 found temperatures of −0.1°±0.1° to prevail below the limit of annual chill, 20 m. below the snow surface. Anti-freeze poured into the Salmon Glacier would have been sufficient in May 1956 to protect against freezing down to a temperature of −6.0° C., and the fact that no part of the pipe had become frozen by August of that year indicated that the glacier at depth was warmer than this temperature.
High hydrostatic pressures within the ice are indicated by scattered data. The drill pipe was filled with a mixture of anti-freeze and water to 99 m. from the top on 5 May 1956 and to 93 m. two days later; on 9 August 1956 the water level was approximately 53 m. from the top. The abrupt rise of water level indicates reasonably free contact with fluids outside the casing. Indications of damage in the lowermost 12 m. of the pipe in August suggests that the fluid within the pipe could be in contact with the outside through breaks at this level. Here the hydrostatic pressure exerted by the fluid column would be almost exactly that exerted by the ice itself.
Records in the other holes are less satisfactory. On 8 May 1956 Hole 2 was filled with water to 28 m. from the surface and Hole 3 to 44 m. from the surface. Both holes, however, had been drilled several weeks previously and were not cased. Both may have been sealed off by a plug of ice and either expansion of the fluid on freezing or the squeezing of the upper part of the hole may have contributed to high water levels.
An estimate of mass transport of this glacier is also possible. Surface velocity at three points on the ice surface averages 91 m./yr. The average velocity in any vertical section, judging from the surveyed hole, is about 85 per cent of the surface velocity, or 77 m./yr. for the glacier as a whole. The area of cross-section of the glacier (Fig. 1) is roughly 975,000 m.2 Thus annual transport of ice alone, omitting any water that may pass this section in the fluid state,Reference Mathews 9 is 75×106 m.3/yr. This is derived from an accumulation area of about 29 km.2 (including a small fraction of bare hillsides which discharge avalanche snow onto the névé) at a rate of 2.6 m.3/m.2yr., or 2.3 m. of water equivalent per year.
Acknowledgements
The writer acknowledges with appreciation the assistance given him in this project by the following organizations: Granduc Mines Limited for the use of the holes, for the installation of the pipe, for transportation and for many courtesies; the Associate Committee on Soil and Snow Mechanics of the National Research Council for financial assistance; the Parsons Survey Company for loan of the inclinometer on three occasions; to the Pacific Naval Laboratory, Esquimalt, B.C., for loan of thermistor cable and electric bridge and the University of British Columbia for purchasing and secretarial help. He wishes too, to express appreciation to the many individuals who also contributed hard work, advice, or both: Messrs. J. Crowhurst, J. Parker, D. Sunderland and T. Harris of Granduc Mines; Dr. G. A. Garland, Messrs. J. Adkins and E. Farar of the University of Toronto geophysical team of 1957, to Mr. Eric Terzaghi, and to Professor R. P. Sharp.