Abstract
Purpose. The main goal of this study was to synthesize and characterize hydrogels which undergo reversible gel-sol phase transformation in response to changes in glucose concentration in the surrounding environment.
Methods. The glucose-sensitive hydrogels were made by mixing the appropriate concentrations of acrylamide-allyl glucose copolymer and concanavalin A (Con A). To examine their phase reversibility, hydrogels in dialysis membranes were cycled between glucose-free and glucose-containing buffers. The binding affinity of allyl glucose (AG) to Con A was examined by using an equilibrium dialysis technique.
Results. The synthesized hydrogels underwent phase transition to sol in the presence of free glucose in the environment. The concentration of external free glucose (Cf) had to be at least 4 times that of polymer-bound glucose (Cp) to induce phase transition from gel to sol. The binding affinity study showed that binding of AG to Con A was four times stronger than that of free glucose. When Cp in the gel was 0.42 mg/ml or higher, Cf had to be much higher than 4 times Cp to induce phase transition.
Conclusions. The synthesized hydrogels underwent phase transition in the presence of free glucose in the environment, but the phase transition was not linearly dependent on the concentration of free glucose. This non-linear dependence was explained by the increased binding affinity of AG over native glucose to Con A, and the cooperative interactions between polymer-bound glucose and Con A.
Similar content being viewed by others
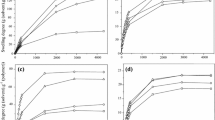
REFERENCES
J. Heller. Chemically self-regulated drug delivery systems, J. Controlled Release 8:111–125 (1988).
M. Brownlee and A. Cerami. A glucose-controlled insulin delivery system: semisynthetic insulin bound to lectin. Science 206:1190–1191 (1979).
S. Y. Jeong, S. W. Kim, M. J. D. Eenink, and J. Feijin. Self-regulating insulin delivery systems I. Synthesis and characterization of glycosylated insulin. J. Controlled Release 1:57–66 (1984).
S. Sato, S. Y. Jeong, J. C. McRea, and S. W. Kim. Self-regulating insulin delivery systems II. In vitro studies. J. Controlled Release 1:67–77 (1984).
J. Kost, T. A. Horbett, B. D. Ratner, and M. Singh. Glucose sensitive membranes containing glucose oxidase: activity, swelling, and permeability studies. J. Biomed. Mater. Res. 19:1117–1133 (1985).
R. A. Siegel. pH-sensitive gels: swelling, equilibria, kinetics, and application for drug delivery. In J. Kost (ed.), Pulsed and self-regulated drug delivery, CRC Press, Boca Raton, FL, 1990, pp. 129–157.
C. K. Kim, E. B. Im, S. J. Lim, Y. K. Oh, and S. K. Han. Development of glucose-triggered pH-sensitive liposomes for a potential insulin delivery. Int. J. Pharm. 101:191–197 (1994).
K. Sawahata, M. Hara, H. Yasunaga, and Y. Osada. Electrically controlled drug delivery systems using polyelectrolyte gels. J. Controlled Release 14:253–262 (1990).
D. S. T. Hsieh, R. Langer, and J. Folkman. Magnetic modulation of release of macromolecules from polymers. Proc. Natl. Acad. Sci. USA 78:1863–1867 (1981).
S. Miyazaki, C. Yokouchi, and M. Tanaka. External control of drug release: controlled release of insulin from a hydrophilic polymer implant by ultrasound irradiation in diabetic rats. J. Pharm. Pharmacol. 40:716–717 (1988).
M. V. Sefton, R. L. Broughton, M. E. Sugamori, and C. L. Mallabone. Hydrophilic polyelectrolytes for the microencapsulation of fibroblasts or pancreatic islets. J. Controlled Release 6:177–187 (1987).
A. M. Sun and G. M. O'Shea. Microencapsulation of living cells-a long-term delivery system. J. Controlled Release 2:137–141 (1985).
H. Uludag, J. E. Babensee, T. Roberts, L. Kharlip, V. Horvath, and M. V. Sefton. Controlled release of dopamine, insulin and other agents from microencapsulated cells. J. Controlled Release 24:3–12 (1993).
P. F. Gores, J. S. Najarian, E. Stephanian, J. J. Lloveras, S. L. Kelley, and D. E. R. Sutherland. Insulin independence in type I diabetes after transplantation of unpurified islets from single donor with 15-deoxyspergualin. Lancet 341:19–21 (1993).
S. J. Lee and K. Park. Synthesis of sol-gel phase-revrsible hydrogels sensitive to glucose. Proc. Intern. Symp. Control. Rel. Bioact. Mater. 21:93–94 (1994).
S. J. Lee and K. Park. Synthesis and characterization of sol-gel phase-reversible hydrogels sensitive to glucose. Polymer Preprints 35(2):391–392 (1994).
S. J. Lee and K. Park. Glucose-sensitive phase-reversible hydrogels. ACS Symposium Series 627:11–16 (1996).
S. J. Lee and K. Park. Synthesis and characterization of sol-gel phase-reversible hydrogels sensitive to glucose. J. Molecular Recognition, in press.
M. J. Taylor, S. Tanna, and G. G. Adams. Insulin delivery system using a novel glucose sensitive formulation. Proc. Intern. Symp. Control. Rel. Bioact. Mater. 22:746–747 (1995).
E. A. Talley, M. D. Vale, and E. Yanovsky. Allyl ethers of carbohydrates III. Ethers of glucose and galactose. J. Am. Chem. Soc. 67:2037–2039 (1945).
W. S. Kim, S. H. Lee, I. K. Kang, and N. K. Park. Release of 8-hydroxyquinoline from copolymers of 8-quinolinyl acrylate and acrylamide. J. Controlled Release 9:281–285 (1989).
M. Fineman and S. D. Ross. Linear method for determining monomer reactivity ratios in copolymerization. J. Polym. Sci. 2:259–262 (1950).
B. B. L. Agrawal and I. J. Goldstein. Protein-carbohydrate interaction VII. Physical and chemical studies on concanavalin A, the hemagglutinin of the jack bean. Arch. Biochem. Biophys. 124:218–229 (1968).
M. Dubois, K. A. Gilles, J. K. Hamilton, P. A. Rebers, and F. Smith. Colorimetric method for determination of sugars and related substances. Anal. Chem. 28:350–356 (1956).
A. J. Kalb and A. Levitski. Metal binding sites of concanavalin A and their role in binding of α-D-glucopyranoside. Biochem. J. 109:669–672 (1968).
G. Scatchard. The attractions of proteins for small molecules and ions. Annal. N. Y. Acad. Sci. 51:660–672 (1949).
L. L. So and I. J. Goldstein. Protein-carbohydrate interaction XX. On the number of binding sites of concanvalin A, the phytohemag-glutinin of the jack bean. Biochim. Biophys. Acta 165:398–404 (1968).
S. Kitano, Y. Koyoma, K. Kataoka, T. Okano, and Y. Sakurai. A novel drug delivery system utilizing a glucose responsive polymer complex between poly(vinyl alcohol) and poly(N-vinyl-2-pyrroli-done) with a phenyl boronic acid moiety. J. Controlled Release 19:162–170 (1992).
J. E. Morris, A. S. Hoffman, and R. R. Fisher. Affinity precipitation of proteins by polyligands. Biotech. Bioeng. 41:991–997 (1993).
K. Nakamae, T. Miyata, A. Jikihara, and A. S. Hoffman. Formation of poly(glycosyloxyethyl methacrylate)-concanavalin A complex and its glucose sensitivity. J. Biomater. Sci. Polym. Edn. 6:79–90 (1994).
J. B. Sumner and S. F. Howell. The identification of the hemagglutinin of the jack bean with concanavalin A. J. Bacteriol. 32:227–237 (1936).
J. L. wang, B. A. Cunningham, and G. M. Edelman. Unusual fragments in the subunit structure of concanavalin A. Proc. Natl. Acad. Sci. USA 68:1124–1130 (1971).
E. Smith and I. J. Goldstein. Protein-carbohydrate interaction V. Further inhibition studies directed toward defining the steriochemical requirements of the reactive sites of concanavalin A. Arch. Biochem. Biophys. 121:88–95 (1967).
P. F. Predki and B. Sarkar. Cooperative interaction of estrogen receptor ‘zinc finger’ domain polypeptides on DNA binding. Biochem. J. 305:805–810 (1995).
S. D. Lewis, J. A. Shafer, and I. J. Goldstein. Kinetic parameters for the binding of p-nitrophenyl-α-D-mannopyranoside to concanavalin A. Arch. Biochem. Biophys. 172:689–695 (1976).
R. D. Farina and R. G. Wilkins. Kinetics of interaction of α-and β-D-monosaccharides with concanavalin A. Biochim. Biophys. Acta 631:428–438 (1980).
V. Horejsi, M. Ticha, and J. Kocourek. Studies on lectins XXXI. Determination of dissociation constants of lectin sugar complexes by means of affinity electrophoresis. Biochim. Biophys. Acta 499:290–300 (1977).
R. D. Poretz and I. J. Goldstein. An examination of the topograghy of the saccharide binding sites of concanavalin A and of the forces involved in complexation. Biochemistry 9:2890–2896 (1970).
Author information
Authors and Affiliations
Rights and permissions
About this article
Cite this article
Obaidat, A.A., Park, K. Characterization of Glucose Dependent Gel-Sol Phase Transition of the Polymeric Glucose-Concanavalin A Hydrogel System. Pharm Res 13, 989–995 (1996). https://doi.org/10.1023/A:1016090103979
Issue Date:
DOI: https://doi.org/10.1023/A:1016090103979