Abstract
Since eighties, 400 and 500 mm thick precast-prestressed concrete hollow core slabs, characterized by increasingly optimized cross-sections with non-circular voids, became very common. However, deeper slabs with long spans, which have to resist high line loads acting close to the supports, are subjected to initial web shear cracking and may fail at loads less than those predicted by traditional codes prescriptions. The shear strength capacity of these members without transverse reinforcement is evaluated through a campaign of detailed nonlinear finite element analyses, matching experimental test data collected from past programs. Constitutive models, based on nonlinear fracture mechanisms, are considered to numerically reproduce the experimental response of single span, simply supported, isolated hollow core units, highlighting web-shear failure mechanism, due to short development length and lack of transverse reinforcement. The adopted diffuse smeared fixed cracking constitutive model allows a reliable prediction of shear stress distributions and crack patterns for these members in their inelastic branch. The presence of a variable inclined strut is clearly evident. Peak shear stress is localized at the bottom side of the cross-section, rather than at the level of the centroid. The experienced brittle web-shear failure mechanism is governed by hollow core shapes with circular or non-circular voids, as evidenced by the evolution of the principal tensile strain distributions. Typically, less inclined, more rounded, diagonal crack, controlled by the smooth web width variation along depth, is opposed to a fairly constant variation of the fracture angle inclination, governed by the abrupt and irregular web width drop.













Similar content being viewed by others
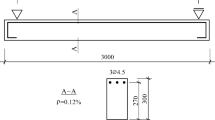
Abbreviations
- a :
-
Shear span
- a 1 :
-
Distance between two line loads
- α 1 :
-
Ratio of distance from end of member to transfer length
- A low :
-
Area of lower strands
- A up :
-
Area of upper strands (if present)
- b w :
-
Sum of web widths
- D :
-
Cross-section depth, assumed as H S
- D i/D i,max :
-
Normalized depth
- D low :
-
Diameter of lower strands
- D up :
-
Diameter of upper strands (if present)
- D z :
-
Mid-span vertical displacement
- d p,low :
-
Average distance from lower strand to the soffit
- δ C50 :
-
Standard deviation of f C50,mean
- E 1 :
-
Principal tensile strain
- E 3 :
-
Principal compressive strain
- F z :
-
Shear force at the support
- f c :
-
Concrete compressive strength
- f C50,mean :
-
Mean concrete compressive strength by cylinder tests on 50 mm cores drilled from the tested specimens
- f ctd :
-
Design tensile strength of concrete per EC2
- f t :
-
Concrete tensile strength
- ϕ :
-
Diameter of strand
- H :
-
Nominal slab depth
- H H :
-
Average depth of hollow core
- H S :
-
Average depth of slab cross-section
- I :
-
Moment of inertia of the section about the centroidal axis
- LF :
-
Normalized load level
- L s :
-
Distance between slab end and centre of support
- L t :
-
Transfer length of prestressing force in strands, assumed as 55ϕ (slow release)
- N low :
-
Number of lower strands
- N up :
-
Number of upper strands (if present)
- R i :
-
Ratio between experimentally observed and predicted shear strength capacity
- S :
-
First area moment of the section about the centroidal axis
- S low :
-
Largest slippage of lower strands
- S up :
-
Largest slippage of upper strands (if present)
- σ cp :
-
Fully effective concrete compressive stress at the centroid due to prestressing
- σ p :
-
Prestress in the strands within the transfer region
- σ p0 :
-
Fully effective prestress in the strands outside the transfer region
- σ p0,low :
-
Initial fully effective prestress in lower strands outside the transfer region
- σ p0,up :
-
Initial fully effective prestress in upper strands (if present) outside the transfer region
- t b :
-
Length of bearing
- t low :
-
Average concrete thickness below the hollow core
- τ yz :
-
Shear stress
- V obs :
-
Experimentally observed shear strength capacity
- V pre :
-
Predicted shear strength capacity
- x :
-
Coordinate along the longitudinal axis of the member
- x/D :
-
Ratio between longitudinal distance from the support and cross-section depth
References
ACI Committee (2005) Building code requirements for structural concrete (ACI 318–05) and commentary (ACI 318R–05). American Concrete Institute, Farmington Hills
Becker RJ, Buettner DR (1985) Shear tests of extruded hollow core slabs. PCI J 30(2):40–54
Canadian Standards Association (2001). CSA standard A23.3-04 design of concrete structures
CEB-FIP (1990) CEB-FIP Model Code. Comité Euro-International du Béton, 1993
Commission for the European Communities (2005) Eurocode 2: design of concrete structures—Part 1: general rules and rules for buildings, EN 1992-1-1
Cladera A, Marí AR (2005) Experimental study on high-strength concrete beams failing in shear. Eng Struct 27(10):1519–1527
Duthinh D (1999) Sensitivity of shear strength of reinforced concrete and prestressed concrete beams to shear friction and concrete softening according to modified compression field theory. ACI Struct J 96(4):495–508
Girhammar UA (1992) Design principles for simply supported prestressed hollow core slabs. Struct Eng Rev, Oxford, England, 4(4): 301–316
Hawkins NM, Ghosh SK (2006) Shear strength of hollow core slabs. PCI J 51(1):110–115
Mpampatsikos V, Nascimbene R, Petrini L (2008) A critical review of the RC frame existing building assessment procedure according to EUROCODE 8 and Italian seismic code. J Earthq Eng 12(S1):52–82
Nascimbene R, Rassati GA, Wijesundara KK (2011) Numerical simulation of gusset-plate connections with rectangular hollow section shape brace under quasi-static cyclic loading. J Constr Steel Res 70:177–189
Pajari M (1989) Design of prestressed hollow core slabs. Research Report 657, VTT Building and Transport, Finland
Pajari M (2005) Resistance of prestressed hollow core slabs against web shear failure. Research Notes 1292, VTT Building and Transport, Finland
Palmer KD, Schultz AE (2011) Experimental investigation of the web-shear strength of deep hollow-core units. PCI J 56(4):83–104
Santagati S, Bolognini D, Nascimbene R (2012) Strain life analysis at low cycle fatigue on concentrically braced steel structures with RHS shape braces. J Earthq Eng 16(S1):107–137
Selby RG, Vecchio FJ (1993) Three-dimensional constitutive relations for reinforced concrete, Technical Report 93–02, University of Toronto, Department Civil Engineering Toronto, Canada
Shahawy MA, Issa M, Batchelor BD (1992) Strand transfer lengths in full scale AASHTO prestressed concrete girders. PCI J 37(3):84–96
Thorenfeldt E, Tomaszewicz A, Jensen JJ (1987) Mechanical properties of high-strength concrete and applications in design. In: Proceedings symposium utilization of high-strength concrete, Trondheim, Norway, Tapir
Vecchio FJ, Collins MP (1986) The modified compression field theory for reinforced concrete elements subjected to shear. ACI Struct J 83(22):219–231
Vecchio FJ, Collins MP (1993) Compression response of cracked reinforced concrete. J Struct Eng ASCE 119(12):3590–3610
Walraven JC, Mercx WPM (1983) The bearing capacity of prestressed hollow core slabs. Heron, 28(3), p. 46
Wijesundara KK, Bolognini D, Nascimbene R, Calvi GM (2009) Review of design parameters of concentrically braced frames with RHS shape braces. J Earthq Eng 13(S1):109–131
Yang L (1994) Design of prestressed hollow core slabs with reference to web shear failure. J Struct Eng ASCE 120(9):2675–2696
Author information
Authors and Affiliations
Corresponding author
Rights and permissions
About this article
Cite this article
Brunesi, E., Bolognini, D. & Nascimbene, R. Evaluation of the shear capacity of precast-prestressed hollow core slabs: numerical and experimental comparisons. Mater Struct 48, 1503–1521 (2015). https://doi.org/10.1617/s11527-014-0250-6
Received:
Accepted:
Published:
Issue Date:
DOI: https://doi.org/10.1617/s11527-014-0250-6