Regulatory Mechanisms Underlying the Differentiation of Neotenic Reproductives in Termites: Partial Release From Arrested Development
- 1Misaki Marine Biological Station, School of Science, The University of Tokyo, Miura, Japan
- 2National Institute of Advanced Industrial Science and Technology, Tsukuba, Japan
- 3Faculty of Science, Academic Assembly, University of Toyama, Gofuku, Japan
Eusocial insects exhibit reproductive division of labor, in which only a part of colony members differentiates into reproductives. In termite colonies, the division of labors is performed among multiple types of individuals (i.e., castes), such as reproductives, workers, and soldiers to organize their society. Caste differentiation occurs according to extrinsic factors, such as social interactions, leading to developmental modifications during postembryonic development, and consequently, the caste ratio in a colony is appropriately coordinated. In particular, when the current reproductives die or become senescent, some immature individuals molt into supplementary reproductives, also known as “neotenics,” that take over the reproductive task in their natal colony. Neotenics exhibit variety of larval features, such as winglessness, and thus, immature individuals are suggested to differentiate by a partial release from arrested development, particularly in the reproductive organs. These neotenic features, which have long been assumed to develop via heterochronic regulation, provide us opportunities to understand the developmental mechanisms and evolutionary origin of the novel caste. This article overviews the accumulated data on the physiological and developmental mechanisms that regulate the neotenic differentiation in termites. Furthermore, the evolutionary trajectories leading to neotenic differentiation are discussed, namely the acquisition of a regulatory mechanism that enable the partial release from a developmentally arrested state.
Introduction: Caste Differentiation in Termites
The acquisition of the caste system in social insects is a critical step in eusocial evolution, in which colony tasks are allocated to different types of individuals with caste-specific morphological features (Lin and Michener, 1972). Regulatory mechanisms underlying the development of caste-specific characteristics should be deeply associated with eusociality (Miura, 2005). In particular, the reproductive division of labor between the reproductive and sterile castes is one of the most distinctive features in eusocial insects (Wilson, 1971, 1975). Among eusocial insects, termites, which are distantly related to hymenopterans (Noirot, 1969; Korb, 2015), exhibit highly sophisticated and complex societies. It is believed that the termite sociality first emerged in the Early Cretaceous, approximately 50 million years before the appearance of eusocial hymenopterans (Grimaldi and Engel, 2005; Roisin and Korb, 2011; Engel, 2015). Although regulatory mechanisms that control the differentiation of reproductive and sterile castes are thought to differ between hymenopterans and termites, most of the involved mechanisms are unclear, particularly in termites.
Generally, in hemimetabolous insects (e.g., cockroaches, locusts, etc.), adult-specific characteristics, such as compound eyes, wings, and reproductive organs, gradually develop during postembryonic development via moltings, and the dramatic morphological modifications occur at the final molt, i.e., imaginal molt (Anderson, 1972). In termites, caste-specific morphological characteristics are created through moltings (Noirot, 1969; Roisin, 2000), in which the developmental patterns differ depending on the caste fate (Miura, 2005; Korb and Hartfelder, 2008). Therefore, the regulatory mechanisms of molting and metamorphosis contribute to caste-specific development. Concerning the differentiation into alates, i.e., winged reproductives that form new colonies, imaginal characteristics, such as compound eyes, wings, and gonads, dramatically develop (Nutting, 1969; Weesner, 1969; Nii et al., 2019). Thus, the differentiation into alates is considered homologous to the imaginal development in other hemimetabolous insects (Nalepa and Bandi, 2000). In particular, termite colonies include numerous sexually immature individuals engaged in various tasks such as nursing and foraging (Thorne, 1996; Korb and Hartfelder, 2008). In these individuals, the development of imaginal organs like compound eyes, wings, and gonads is restricted or arrested during postembryonic development (Nalepa and Bandi, 2000; Bourguignon et al., 2016).
As caste differentiation fates are determined during postembryonic development in termites, the patterns of caste differentiation are often depicted as differentiation pathways. Among termite families, two major patterns of caste differentiation pathways are recognized, i.e., linear and bifurcated pathways (Figure 1; Noirot, 1969; Roisin, 2000; Bourguignon et al., 2014). Bifurcated pathways have been described in Mastotermitidae, Hodotermitidae, Termitidae, and some species of Rhinotermitidae, in which the differentiation point for alates and apterous individuals occurs at a relatively early stage of postembryonic development, i.e., first or second larval instars (Roisin, 2000). Workers in the bifurcated pathways cannot develop into alates, and thus, they are called “true workers,” although they still have the potential to develop into reproductives (Korb and Hartfelder, 2008; Bourguignon et al., 2016). In contrast, in the linear pathways observed in Archotermopsidae, Stolotermitidae, Kalotermitidae, and Serritermitidae, in addition to some species of Rhinotermitidae, elder larval individuals serve as “workers” while possessing the potential to differentiate into alates, and thus, they are called pseudergates, meaning “false workers” (Grassé and Noirot, 1947; Korb and Hartfelder, 2008).
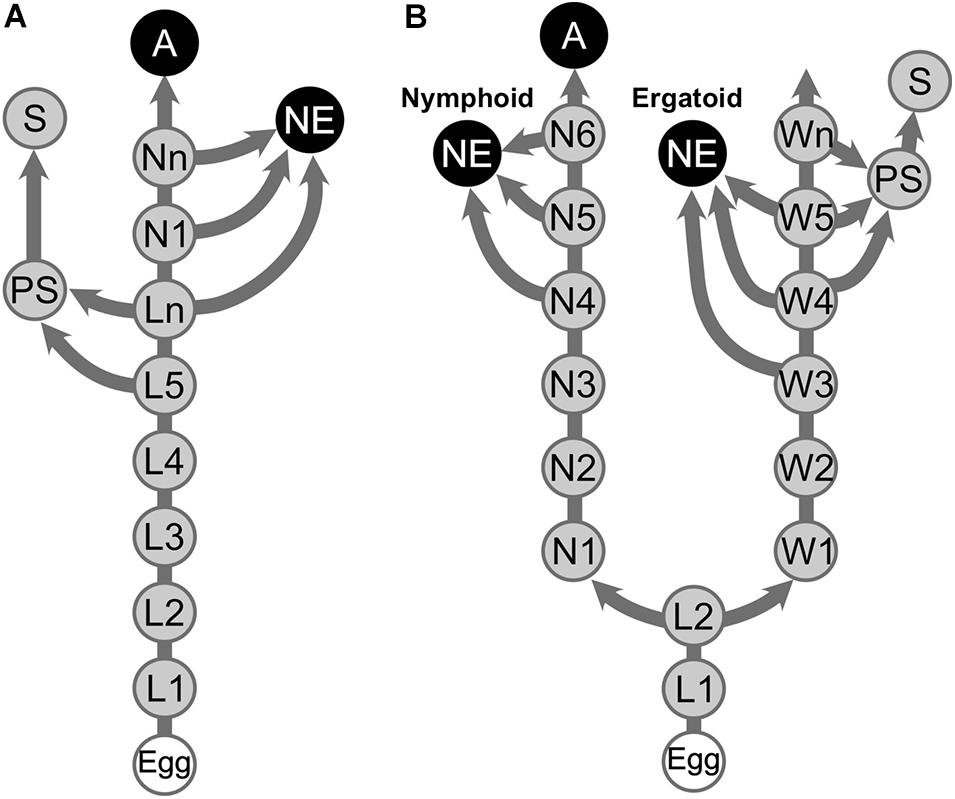
Figure 1. Representative caste developmental patterns in termites. (A) Linear pathway. (B) Bifurcated pathway. Arrows indicate hatching and molts. A, Alate; L, Larva; N, Nymph; NE, Neotenic reproductive; PS, Presoldier; S, Soldier; W, Worker. Elder larvae (Ln) in the linear pathway are referred to as “pseudergates” in this article.
In addition to these developmental stages, other derived pathways, i.e., soldier and neotenic differentiation pathways, have also been investigated by many researchers. Both soldier and neotenic castes are novel developmental stages only present in termites, and these castes represent synapomorphies of the termite lineage, as they are present in all termite species with few exceptions (Noirot, 1969; Korb, 2015). Soldiers differentiate from workers or pseudergates through two molting events via a presoldier stage (Noirot, 1969; Roisin, 2000; Korb and Hartfelder, 2008). Because soldier differentiation can be artificially induced by the application of juvenile hormone (JH), several studies have described soldier developmental process in different species (Hrdý and Křeček, 1972; Howard and Haverty, 1979; Scharf et al., 2003). During soldier differentiation, the body plan of termites, particularly the anterior parts that are used for defensive behaviors, are largely modified through a specific morphogenesis (Miura and Matsumoto, 2000; Koshikawa et al., 2003; Watanabe and Maekawa, 2008; Toga et al., 2013). The morphogenetic process occurs downstream of hormonal and morphogenic factors, which are well documented (Cornette et al., 2008; Sugime et al., 2019; Miura and Maekawa, 2020). However, until recently, because methods for inducing neotenic differentiation had not been established, studies on neotenic differentiation are relatively sparse compared with those of soldier differentiation (Shimoji et al., 2017; Oguchi et al., 2020).
Neotenic reproductives are seen in nearly all the termite families, although they are occasionally lost in some genera (Myles, 1999; Bourguignon et al., 2016). Neotenics, also known as secondary reproductives, can also be referred to as replacement or supplementary reproductives, depending on situations of occurrence (Korb and Hartfelder, 2008). At the time when primary reproductives die or become senescent, they differentiate from immature individuals and exclusively engage in reproduction in their natal nests (Figure 1; Lüscher, 1961; Thorne, 1996; Korb and Hartfelder, 2008). Some species produce neotenics not only as replacements but also as backup against the loss of primary reproductives (Matsuura et al., 2009; Hellemans et al., 2019). Upon the differentiation of neotenic reproductives, adult-specific characteristics related to reproductive organs develop, whereas other imaginal features, such as wings, do not (Figure 2). Thus, it is suggested that the neotenic-specific morphology is accomplished by a heterochronic regulation, in which the development is accelerated or arrested depending on body modules (Nalepa and Bandi, 2000).
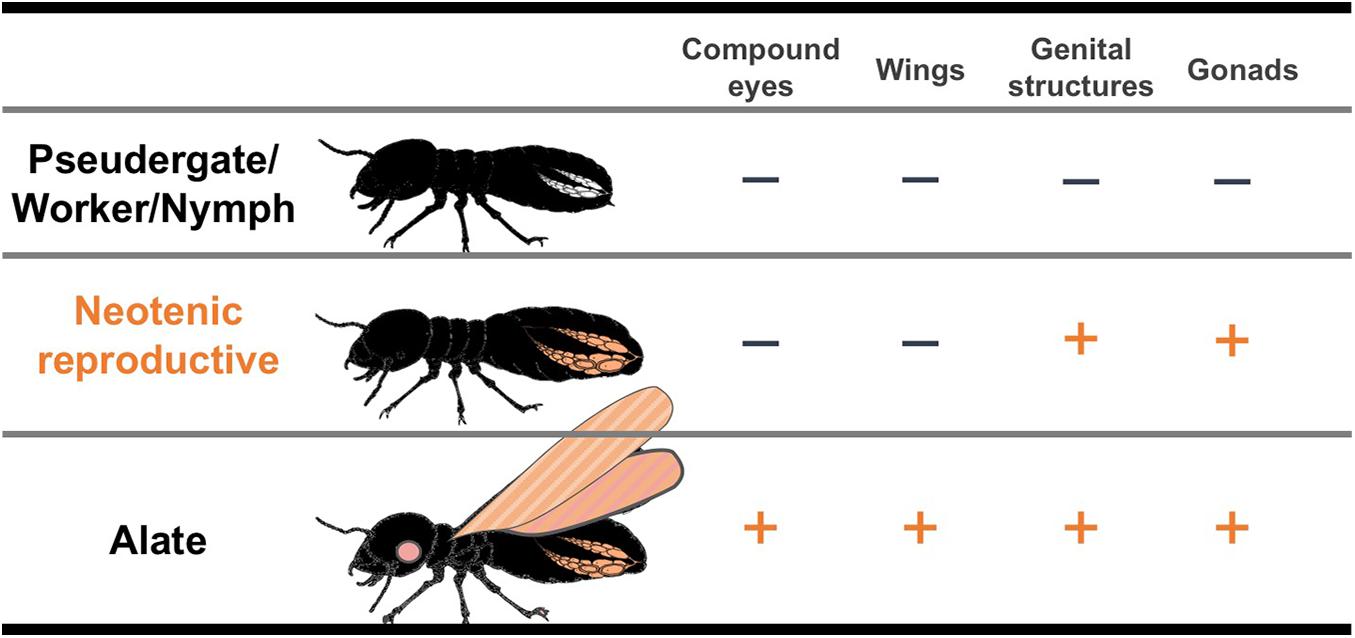
Figure 2. Schematic illustrations of the developmental degree of imaginal organs in each caste. “+” indicates well-developed imaginal organs like alates. “–” indicates imaginal organs with suppressed or interrupted developments compared with those of alates.
The differentiation of neotenic reproductives is controlled by genetic, maternal, and environmental factors. In Reticulitermes speratus and several other species, for instance, genetic, and maternal factors influenced by sex-linked genetic mechanisms, i.e., the asexual queen succession (AQS) system, are responsible for the neotenic differentiation (Hayashi et al., 2007; Matsuura et al., 2009; Matsuura et al., 2018; Hellemans et al., 2019), although environmental factors during the postembryonic development also affect the caste fate determination (Hayashi et al., 2007, 2013). In contrast, environmental factors during postembryonic development, such as social interactions between reproductives and non-reproductive castes, are also determinant force for the neotenic differentiation (Lüscher, 1974; Shimoji et al., 2017; Sun et al., 2017; Masuoka et al., 2021). Recently, neotenic differentiation was studied by applying induction methods in species with bifurcated and linear caste differentiation pathways (Saiki and Maekawa, 2011; Shimoji et al., 2017), focusing on morphogenetic processes during differentiation and the underlying physiological regulation (Saiki et al., 2015; Oguchi and Miura, 2019; Oguchi et al., 2020). By reviewing accumulated knowledge on the physiological and developmental regulation of neotenic differentiation in termites, the evolutionary processes toward the acquisition of neotenic reproductives are hypothesized.
Modularity: Reproductive Organ-Specific Morphogenesis
Neotenic reproductives in termites exhibit the juvenile characteristics of hemimetabolous insects, and thus, they are termed “neotenic” (Thorne, 1996). Meanwhile, they lack imaginal characteristics such as well-developed wings and compound eyes (Maekawa et al., 2008; Saiki and Maekawa, 2011). Neotenics can be roughly classified into two types: “nymphoids” that differentiate from nymphs and “ergatoids” that differentiate from workers (Korb and Hartfelder, 2008). Depending on the developmental stages from which neotenics derive, the developmental degree of imaginal characters differs (e.g., nymphoids have wing buds and slightly developed eyes, whereas ergatoids lack these features). However, in all cases, neotenics possess well-developed gonads that are comparable to those of primary reproductives (Thorne, 1996; Korb and Hartfelder, 2008; Saiki and Maekawa, 2011; Oguchi et al., 2016). This suggests that gonadal development is accelerated in neotenics in contrast to other body parts. This acceleration might be a case of “paedomorphosis,” which is a type of heterochrony that involves a delay of specific organ development (Reilly et al., 1997; Nalepa and Bandi, 2000). The adaptive significance of neotenic differentiation, in which only some imaginal characters develop, might be that it permits individuals to immediately become reproductives through fewer moltings. In most cases, one molting event is required for neotenic differentiation from the previous undifferentiated stages, such as pseudergates, workers, or nymphs (Korb and Hartfelder, 2008), except in some species with bifurcated caste differentiation pathways of Reticulitermes labralis, Nasutitermes corniger, and Nasutitermes aquilinus, in which the pre-neotenic stages and two molts are required (Thorne and Noirot, 1982; Su et al., 2017; da Silva et al., 2019). The fact that the neotenic differentiation requires moltings suggests that morphological alterations are required for reproductive activities such as copulation and oviposition. In the case of neotenic molt (only female individuals) in a dampwood termite Hodotermopsis sjostedti, which possesses a linear caste differentiation pathway, the loss of styli at the abdominal tip and expansion of the seventh sternites are observed (Oguchi and Miura, 2019). Therefore, the differentiation of neotenic reproductives may be accomplished by a partial release of immature individuals from arrested development, particularly in the reproductive organs. In other words, the pre-existing imaginal developmental processes (i.e., a late differentiation processes) are partially involved in neotenic differentiation; therefore, neotenic differentiation may be regarded as a case of “modular heterochrony.” Thus, the neotenic reproductive is a distinctive phenotype (caste) that morphologically differs from primary reproductives (alates) (Figure 2). These mosaic-like phenotypes were not only observed in termites but also in eusocial hymenopteran species, particularly in ants (Wheeler and Nijhout, 1981; Abouheif and Wray, 2002; Miyazaki et al., 2010; Yang and Abouheif, 2011). Of note, “ergatoid queen” in ants, which has both the queen and worker traits, was believed to have emerged from the combination of the caste developmental systems (Miyazaki et al., 2010; Yang and Abouheif, 2011; Molet et al., 2012). Therefore, modular and heterochronic regulation by tinkering with the pre-existing developmental systems are thought to be important and ubiquitous evolutionary mechanisms for the acquisition of novel castes (cf. Cohen, 1999).
Heterochronic Shifts via Physiological Control
Generally in insects, molting and metamorphosis are coordinated by JH and molting hormone (i.e., ecdysone) (Belles and Santos, 2014). It is well known that the imaginal molt is induced by the lowering of JH titers in an insect (Romaña et al., 1995; Treiblmayr et al., 2006). A similar physiological pattern is also present during alate differentiation in termites (Figure 3A; Nijhout and Wheeler, 1982; Cornette et al., 2008). However, detailed physiological analyses, especially on JH dynamics, have yet to be conducted concerning the differentiation into neotenic reproductives, although previous studies predicted that the shift from low to high JH titers during the intermolt period would induce neotenic differentiation (reviewed in Nijhout and Wheeler, 1982; Korb, 2015). Some studies actually reported an extraordinary high JH titer in the female neotenics (e.g., Elliott and Stay, 2007; Maekawa et al., 2010; Korb et al., 2012; Saiki et al., 2015). Similar tendencies of high JH titers were observed after female alate differentiation (Cornette et al., 2008; Maekawa et al., 2010). The high JH titers in female reproductives probably contribute to the development of female reproductive systems, including ovaries (Korb, 2015; Saiki et al., 2015). Furthermore, in many insects including termites, JH is sequestered in fat bodies and engaged in yolk protein synthesis (Nijhout, 1994), suggesting that this function of JH is also driven at the time of neotenic differentiation. However, the JH function in the ovarian development is only applicable to the differentiation of female neotenics, and thus, the JH action leading to the differentiation of male neotenics should also be elucidated in future studies.
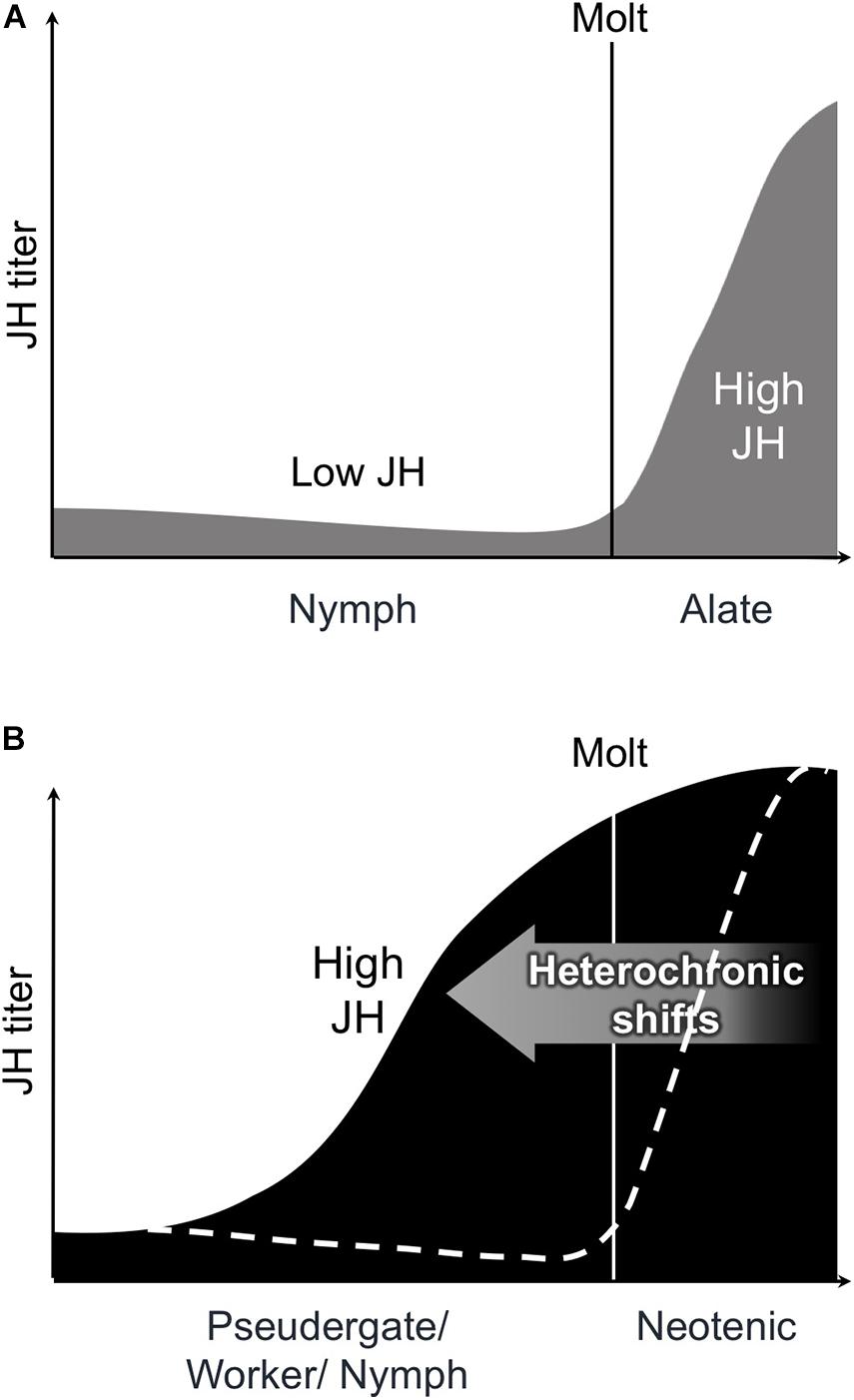
Figure 3. Schematic illustrations of models on the transitions of juvenile hormone (JH) titer (vertical axis) during alate or neotenic differentiation. (A) Consistently-lowered JH titer lead alate molt from nymphal instar. After alate molt, the JH titer rise in female alates. Figures were modified as per Cornette et al. (2008, Figure 7). (B) JH titer ransition from low to high leads the neotenic differentiation. Dotted line indicates the JH titer during alate molt.
Recently, the physiological regulation at the time of neotenic differentiation was unraveled in the dampwood termite H. sjostedti (Oguchi et al., 2020). This research applied an induction method for neotenic differentiation in which the sex caste ratio was artificially manipulated (Shimoji et al., 2017). Under these experimental conditions, the existence of only a male (or female) neotenic reproductive promotes the differentiation of female (or male) neotenics from pseudergates. Their findings illustrated that the JH titer of female pseudergates in the presence of a male neotenic without a female neotenic continuously lowered and then increased immediately before the molt (Oguchi et al., 2020). Thus, this study supports the hypothesis by Nijhout and Wheeler (1982), at least in terms of the female neotenic differentiation, considering that neotenic differentiation requires a transition of JH titers from low to high (Figure 3B). The similar tendencies and models have been shown in other species, such as R. speratus (Saiki et al., 2015) and Cryptotermes secundus (Korb et al., 2012), suggesting that this model can be applicable to other termite lineages, at least lower termite species. Moreover, the JH analog application of female pseudergates inhibits the reproductive organ development and neotenic molt (Oguchi et al., 2020). This role of JH is similar to the antimetamorphic action of insects and lowering JH titer, which are required for the imaginal molts (Nijhout, 1994). Therefore, the fact that female neotenic differentiation requires low JH titers suggests that the neotenic molt can be regarded as a type of imaginal molt and rapid change of JH titers might be heterochronic shifts (Figures 3A,B). In other words, the neotenic reproductive can be regarded as a “physiological imago.” Recently, the master regulatory pathway controlling molting and metamorphosis, i.e., the MEKRE93 or Met-Krh1-E93 pathway, was demonstrated to work downstream of JH and ecdysone in insects (Belles and Santos, 2014; Ureña et al., 2014), suggesting that this pathway is also involved in caste regulation in termites (Korb and Belles, 2017; Miura and Maekawa, 2020).
Evolutionary Implications
One of the most important steps in the evolution of eusociality is the acquisition of reproductive labor division (Wilson, 1971; Lin and Michener, 1972). In termites, especially in basal species with linear caste pathways, immature individuals, that are homologous to “nymphs” in general hemimetabolous insects, engage in colony tasks without participating in reproduction (Korb and Hartfelder, 2008). These immature individuals undergo successive moltings, but they do not completely develop adult-specific characteristics such as compound eyes and wings (Miura et al., 2004; Katoh et al., 2007; Nii et al., 2019). Considering the evolutionary process of termite eusociality, the appearance of immature castes that are engaged in colony tasks (i.e., pseudergates) would be an important step (cf. Nalepa and Bandi, 2000). Before the emergence of such immature castes, bifurcation (i.e., develop or less develop) of imaginal organ development within the colony members is suggested to lead the reproductive division of labor (Bourguignon et al., 2016). Therefore, during the eusocial evolution in termites, it is suggested that the postembryonic development of hemimetabolous insects is partially (i.e., body parts specifically) or completely arrested during the linear adult developmental process (Bourguignon et al., 2016).
Thus, what mechanisms enable neotenic wingless reproductive differentiation? Some studies provided circumstantial evidences that the development of adult-specific characteristics (e.g., reproductive organs, wings, compound eyes, sensillae) is connected and induced under the control of physiological signals (i.e., hormonal signals), leading to alate differentiation (e.g., Miura and Matsumoto, 1996). Therefore, during neotenic differentiation, such alate-specific characteristics could also be expressed. In fact, neotenic reproductives display dark coloration of the cuticles caused by pigmentation and sclerotization during differentiation that resembles alate coloration (e.g., Watson and Abbey, 1985; Hu and Forschler, 2012). Moreover, it was reported that ergatoids possessed wing bud-like structures on their thoraces and slightly developed compound eyes on their heads (e.g., Noirot and Thorne, 1988; Miura and Matsumoto, 1996; da Silva et al., 2019). This phenomenon suggests that the development of these adult-specific characteristics is interlinked, permitting their expression simultaneously with the gonadal development required for the differentiation of functional ergatoids. These links among adult-specific characteristics are assumed to arise from the linkage of gene regulatory networks.
Recently, transcriptomic analysis suggested that Ras-MAPK pathway genes involved in ergatoid differentiation in Reticulitermes labralis (Ye et al., 2019). In general, the Ras-MAPK pathway is known to control the signal transduction from plasma membrane to nucleus, regulating downstream genes involved in cell proliferation, differentiation, and cell death (Foster and Malek, 2016). Therefore, specific regulations in such cellular processes may be crucial for ergatoid differentiation. It is unclear whether these gene expression changes also occur during nymphoid differentiation; however, the differences of gene expression patterns between ergatoids and nymphoids may help to understand the genetic networks that regulate body part-specific development.
As mentioned previously, there are several morphological and functional differences between alates and neotenics, and thus, there should be different physiological regulatory mechanisms underlying the differentiation processes leading to the two reproductive types. In both alate and neotenic differentiation, JH and ecdysone pathways might be involved in each molt, although the timing of JH elevation differs between them. Therefore, these gaps might explain the partial differences between alates and neotenics. During neotenic differentiation, only some body parts and organs that are related to reproduction undergo further developmental processes that also occur during alate differentiation (Figure 2). In soldier differentiation in termites, such body part-specific morphogenesis, i.e., mandibular enlargement (Koshikawa et al., 2003; Watanabe and Maekawa, 2008; Watanabe et al., 2014; Miura and Maekawa, 2020), is organized by patterning genes providing spatial information, such as Hox genes, which are upregulated under the control of hormonal factors (e.g., Toga et al., 2013; Sugime et al., 2019). Considering these mechanisms, neotenic differentiation should also require similar regulatory mechanisms of gene expression including toolkit genes such as Hox genes and hormonal factors. Obviously, because anterior body parts are dramatically modified during soldier differentiation whereas posterior body parts such as reproductive traits develop during neotenic differentiation, different Hox genes should be responsible for the body-part development in each caste. During the evolutionary processes of the termite lineage, it is suggested that such regulatory mechanisms leading to body part-specific differentiation processes were acquired in the common ancestor, resulting in the appearances of novel castes, such as soldiers and neotenic reproductives. Thus, comparative approaches between termites and sister-group cockroaches, the genus Cryptocercus, may uncover the underpinnings of social evolution in termites.
Author Contributions
KO, KM, and TM wrote the manuscript. All authors read and approved the manuscript. All authors contributed to the article and approved the submitted version.
Funding
This work was supported by a Grant-in-Aid for Scientific Research A (No. 25251041) for TM and a Grant-in-Aid for JSPS Fellows for KO (No. 17J06879) from the Ministry of Education, Culture, Sports, Science and Technology of Japan.
Conflict of Interest
The authors declare that the research was conducted in the absence of any commercial or financial relationships that could be construed as a potential conflict of interest.
Acknowledgments
We would like to thank Dr. Rui Fernandes for giving us the opportunity to write this review. We express our gratitude to Y. Masuoka for the helpful discussions during the redaction of the manuscript. Thanks are also due to M. Hashimoto for helping draw the illustrations.
References
Abouheif, E., and Wray, G. A. (2002). Evolution of the gene network underlying wing polyphenism in ants. Science 297, 249–252. doi: 10.1126/science.1071468
Anderson, D. T. (1972). “The development of hemimetabolous insects,” in Developmental Systems: Insects, Vol. 1, eds S. J. Counce and C. H. Waddington (New York, NY: Academic Press).
Belles, X., and Santos, C. G. (2014). The MEKRE93 (Methoprene tolerant-Krüppel homolog 1-E93) pathway in the regulation of insect metamorphosis, and the homology of the pupal stage. Insect Biochem. Mol. Biol. 52, 60–68. doi: 10.1016/j.ibmb.2014.06.009
Bourguignon, T., Chisholm, R. A., and Evans, T. A. (2016). The termite worker phenotype evolved as a dispersal strategy for fertile wingless individuals before eusociality. Am. Nat. 187, 372–387. doi: 10.1086/684838
Bourguignon, T., Lo, N., Cameron, S. L., Šobotník, J., Hayashi, Y., Shigenobu, S., et al. (2014). The evolutionary history of termites as inferred from 66 mitochondrial genomes. Mol. Biol. Evol. 32, 406–421. doi: 10.1093/molbev/msu308
Cornette, R., Gotoh, H., Koshikawa, S., and Miura, T. (2008). Juvenile hormone titers and caste differentiation in the damp-wood termite Hodotermopsis sjostedti (Isoptera, Termopsidae). J. Insect Physiol. 54, 922–930. doi: 10.1016/j.jinsphys.2008.04.017
da Silva, I. B., Haifig, I., Vargo, E. L., Casarin, F. E., da Mota, M. L., Lima, J. T., et al. (2019). Ergatoid reproductives in the Neotropical termite Nasutitermes aquilinus (Holmgren)(Blattaria: Isoptera: Termitidae): developmental origin, fecundity, and genetics. Insect Sci. 27, 1322–1333. doi: 10.1111/1744-7917.12727
Elliott, K. L., and Stay, B. (2007). Juvenile hormone synthesis as related to egg development in neotenic reproductives of the termite Reticulitermes flavipes, with observations on urates in the fat body. Gen. Comp. Endocrinol. 152, 102–110. doi: 10.1016/j.ygcen.2007.03.003
Foster, S. A., and Malek, S. (2016). The RAS/MAPK axis gets stressed out. Mol. Cell. 64, 854–855. doi: 10.1016/j.molcel.2016.11.024
Grassé, P. P., and Noirot, C. (1947). Le polymorphisme social du termite à cou jaune (Calotermes flavicollis F.). Les faux-ouvriers ou pseudergates et les mues régressives. C. R. Acad. Sci. 224, 219–221.
Grimaldi, D., and Engel, M. S. (2005). The Insects. Evolution of the Insects. Cambridge: Cambridge University Press, 119–147.
Hayashi, Y., Lo, N., Miyata, H., and Kitade, O. (2007). Sex-linked genetic influence on caste determination in a termite. Science 318, 985–987. doi: 10.1126/science.1146711
Hayashi, Y., Miyata, H., Kitade, O., and Lo, N. (2013). Neotenic reproductives influence worker caste differentiation in the termite Reticulitermes speratus (Isoptera; Rhinotermitidae). Sociobiology 60, 446–452.
Hellemans, S., Dolejšová, K., Křivánek, J., Fournier, D., Hanus, R., and Roisin, Y. (2019). Widespread occurrence of asexual reproduction in higher termites of the Termes group (Termitidae: Termitinae). BMC Evol. Biol. 19:131. doi: 10.1186/s12862-019-1459-3
Howard, R. W., and Haverty, M. I. (1979). Termites and juvenile hormone analogues: a review of methodology. Sociobiology 4, 277–285.
Hrdý, I., and Křeček, J. (1972). Development of superfluous soldiers induced by juvenile hormone analogues in the termite, Reticulitermes lucifugus santonensis. Insectes Soc. 19, 105–109. doi: 10.1007/bf02224728
Hu, J., and Forschler, B. T. (2012). Neotenic phenotype and sex ratios provide insight into developmental pathways in Reticulitermes flavipes (Isoptera: Rhinotermitidae). Insects 3, 538–552. doi: 10.3390/insects3020538
Katoh, H., Matsumoto, T., and Miura, T. (2007). Alate differentiation and compound-eye development in the dry-wood termite Neotermes koshunensis (Isoptera, Kalotermitidae). Insectes Soc. 54, 11–19. doi: 10.1007/s00040-006-0900-y
Korb, J. (2015). “Juvenile hormone, a central regulator of termite caste polyphenism,” in Advances in Insect Physiology, Vol. 48, eds A. Zayed and C. F. Kent (Oxford: Elsevier), 132–161.
Korb, J., and Belles, X. (2017). Juvenile hormone and hemimetabolan eusociality: a comparison of cockroaches with termites. Curr. Opin. Insect Sci. 22, 109–116. doi: 10.1016/j.cois.2017.06.002
Korb, J., and Hartfelder, K. (2008). Life history and development – a framework for understanding the ample developmental plasticity in lower termites. Biol. Rev. 83, 295–313. doi: 10.1111/j.1469-185X.2008.00044.x
Korb, J., Hoffmann, K., and Hartfelder, K. (2012). Molting dynamics and juvenile hormone titer profiles in the nymphal stages of a lower termite, Cryptotermes secundus (Kalotermitidae)–signatures of developmental plasticity. J. Insect Physiol. 58, 376–383. doi: 10.1016/j.jinsphys.2011.12.016
Koshikawa, S., Matsumoto, T., and Miura, T. (2003). Mandibular morphogenesis during soldier differentiation in the damp-wood termite Hodotermopsis sjoestedti (Isoptera: Termopsidae). Naturwissenschaften 90, 180–184. doi: 10.1007/s00114-003-0408-5
Lüscher, M. (1961). “Social control of polymorphism in termites,” in Insect Polymorphism, ed. J. S. Kennedy (London: Royal Entomological Society of London), 57–67.
Lüscher, M. (1974). “Kasten und kastendifferenzierung bei niederen termiten,” in Sozialpolymorphismus Bei Insekten, ed. G. H. Schmidt (Stuttgart: Wissenschaftliche Verlagsgesellschaft), 694–739.
Maekawa, K., Ishitani, K., Gotoh, H., Cornette, R., and Miura, T. (2010). Juvenile hormone titre and vitellogenin gene expression related to ovarian development in primary reproductives compared with nymphs and nymphoid reproductives of the termite Reticulitermes speratus. Physiol. Entomol. 35, 52–58. doi: 10.1111/j.1365-3032.2009.00711.x
Maekawa, K., Mizuno, S., Koshikawa, S., and Miura, T. (2008). Compound eye development during caste differentiation of the termite Reticulitermes speratus (Isoptera: Rhinotermitidae). Zoolog. Sci. 25, 699–705. doi: 10.2108/zsj.25.699
Masuoka, Y., Nuibe, K., Hayase, N., Oka, T., and Maekawa, K. (2021). Reproductive soldier development is controlled by direct physical interactions with reproductive and soldier termites. Insects 12:76. doi: 10.3390/insects12010076
Matsuura, K., Mizumoto, N., Kobayashi, K., Nozaki, T., Fujita, T., Yashiro, T., et al. (2018). A genomic imprinting model of termite caste determination: not genetic but epigenetic inheritance influences offspring caste fate. Am. Nat. 191, 677–690. doi: 10.1086/697238
Matsuura, K., Vargo, E. L., Kawatsu, K., Labadie, P. E., Nakano, H., Yashiro, T., et al. (2009). Queen succession through asexual reproduction in termites. Science 323, 1687–1687. doi: 10.1126/science.1169702
Miura, T. (2005). Developmental regulation of caste-specific characters in social-insect polyphenism. Evol. Dev. 7, 122–129. doi: 10.1111/j.1525-142x.2005.05014.x
Miura, T., Koshikawa, S., Machida, M., and Matsumoto, T. (2004). Comparative studies on alate wing formation in two related species of rotten-wood termites: Hodotermopsis sjostedti and Zootermopsis nevadensis (Isoptera: Termopsidae). Insectes Soc. 51, 247–252.
Miura, T., and Maekawa, K. (2020). The making of the defensive caste: physiology, development and evolution of the soldier differentiation in termites. Evol. Dev. 22:425–437. doi: 10.1111/ede.12335
Miura, T., and Matsumoto, T. (1996). Ergatoid reproductives in Nasutitermes takasagoensis (Isoptera: Termitidae). Sociobiology 27, 223–238.
Miura, T., and Matsumoto, T. (2000). Soldier morphogenesis in a nasute termite: discovery of a disk-like structure forming a soldier nasus. Proc. Biol. Sci. 267, 1185–1189. doi: 10.1098/rspb.2000.1127
Miyazaki, S., Murakami, T., Kubo, T., Azuma, N., Higashi, S., and Miura, T. (2010). Ergatoid queen development in the ant Myrmecina nipponica: modular and heterochronic regulation of caste differentiation. Proc. Biol. Sci. 277, 1953–1961. doi: 10.1098/rspb.2010.0142
Molet, M., Wheeler, D. E., and Peeters, C. (2012). Evolution of novel mosaic castes in ants: modularity, phenotypic plasticity, and colonial buffering. Am. Nat. 180, 328–341. doi: 10.1086/667368
Myles, T. G. (1999). Review of secondary reproduction in termites (Insecta: Isoptera) with comments on its role in termite ecology and social evolution. Sociobiology 33, 1–43.
Nalepa, C. A., and Bandi, C. (2000). “Characterizing the ancestors: paedomorphosis and termite evolution,” in Termites: Evolution, Sociality, Symbioses, Ecology, eds T. Abe, D. E. Bignell, and M. Higashi (Dordrecht: Kluwer Publishing House Academic Publishers), 53–76. doi: 10.1007/978-94-017-3223-9_3
Nii, R., Oguchi, K., Shinji, J., Koshikawa, S., and Miura, T. (2019). Reduction of a nymphal instar in a dampwood termite: heterochronic shift in the caste differentiation pathways. EvoDevo 10:10.
Nijhout, H. F., and Wheeler, D. E. (1982). Juvenile hormone and the physiological basis of insect polymorphisms. Q. Rev. Biol. 57, 109–134. doi: 10.1086/412671
Noirot, C. (1969). “Formation of castes in the higher termites,” in Biology of Termites, Vol I, eds K. Krishna and F. M. Weesner (New York, NY: Academic Press), 311–350. doi: 10.1016/b978-0-12-395529-6.50014-3
Noirot, C., and Thorne, B. L. (1988). Ergatoid reproductives in Nasutitermes columbicus (Isoptera, Termitidae). J. Morphol. 195, 83–93. doi: 10.1002/jmor.1051950108
Nutting, W. L. (1969). “Flight and colony foundation,” in Biology of Termites, Vol I, eds K. Krishna and F. M. Weesner (New York, NY: Academic Press), 233–282. doi: 10.1016/b978-0-12-395529-6.50012-x
Oguchi, K., and Miura, T. (2019). Unique morphogenesis in the damp-wood termite: abscission of the stylus during female reproductive caste differentiation. Zoolog. Sci. 36, 380–386. doi: 10.2108/zs190056
Oguchi, K., Shimoji, H., Hayashi, Y., and Miura, T. (2016). Reproductive organ development along the caste differentiation pathways in the dampwood termite Hodotermopsis sjostedti. Insectes Soc. 63, 519–529. doi: 10.1007/s00040-016-0495-x
Oguchi, K., Sugime, Y., Shimoji, H., Hayashi, Y., and Miura, T. (2020). Male neotenic reproductives accelerate additional differentiation of female reproductives by lowering JH titer in termites. Sci. Rep. 10:9435.
Reilly, S. M., Wiley, E. O., and Meinhardt, D. J. (1997). An integrative approach to heterochrony: the distinction between interspeci¢c and intraspecifc phenomena. Biol. J. Linn. Soc. 60, 119–143.
Roisin, Y. (2000). “Diversity and evolution of caste patterns,” in Diversity and Evolution of Caste Patterns, eds T. Abe, D. E. Bignell, and M. Higashi (Dordrecht: Kluwer Academic Publishers), 95–119. doi: 10.1007/978-94-017-3223-9_5
Roisin, Y., and Korb, J. (2011). “Social organisation and the status of workers in termites,” in Biology of Termites: A Modern Synthesis, eds D. E. Bignell, Y. Roisin, and N. Lo (Dordrecht: Springer), 133–164. doi: 10.1007/978-90-481-3977-4_6
Romaña, I., Pascual, N., and Belles, X. (1995). The ovary is a source of circulating ecdysteroids in Blattella germanica (L.) (Dictyoptera, Blattellidae). Eur. J. Entomol. 92, 93–103.
Saiki, R., Gotoh, H., Toga, K., Miura, T., and Maekawa, K. (2015). High juvenile hormone titre and abdominal activation of JH signalling may induce reproduction of termite neotenics. Insect Mol. Biol. 24, 432–441. doi: 10.1111/imb.12169
Saiki, R., and Maekawa, K. (2011). Imaginal organ development and vitellogenin gene expression changes during the differentiation of nymphoids of the termite Reticulitermes speratus. Sociobiology 58
Scharf, M. E., Wu-Scharf, D., Pittendrigh, B. R., and Bennett, G. W. (2003). Caste-and development-associated gene expression in a lower termite. Genome Biol. 4:R62.
Shimoji, H., Oguchi, K., Hayashi, Y., and Miura, T. (2017). Regulation of neotenic differentiation through direct physical contact in the dampwood termite Hodotermopsis sjostedti. Insectes Soc. 64, 393–401. doi: 10.1007/s00040-017-0562-y
Su, X., Yang, X., Li, J., Xing, L., Liu, H., and Chen, J. (2017). The transition path from female workers to neotenic reproductives in the termite Reticulitermes labralis. Evol. Dev. 19, 218–226. doi: 10.1111/ede.12229
Sugime, Y., Oguchi, K., Gotoh, H., Hayashi, Y., Matsunami, M., Shigenobu, S., et al. (2019). Termite soldier mandibles are elongated by dachshund under hormonal and Hox gene controls. Development 146:dev171942. doi: 10.1242/dev.171942
Sun, Q., Haynes, K. F., Hampton, J. D., and Zhou, X. (2017). Sex-specific inhibition and stimulation of worker-reproductive transition in a termite. Sci. Nat. 104:79. doi: 10.1007/s00114-017-1501-5
Thorne, B. L., and Noirot, C. (1982). Ergatoid reproductives in Nasutitermes corniger (Motschulsky)(Isoptera: Termitidae). Int. J. Insect Morphol. Embryol. 11, 213–226. doi: 10.1016/s0020-7322(82)80006-8
Toga, K., Saiki, R., and Maekawa, K. (2013). Hox gene deformed is likely involved in mandibular regression during presoldier differentiation in the nasute termite Nasutitermes takasagoensis. J. Exp. Zool. B Mol. Dev. Evol. 320, 385–392. doi: 10.1002/jez.b.22512
Treiblmayr, K., Pascual, N., Piulachs, M. D., Keller, T., and Belles, X. (2006). Juvenile hormone titer versus juvenile hormone synthesis in female nymphs and adults of the German cockroach, Blattella germanica. J. Insect Sci. 6:43.
Ureña, E., Manjón, C., Franch-Marro, X., and Martín, D. (2014). Transcription factor E93 specifies adult metamorphosis in hemimetabolous and holometabolous insects. Proc. Natl. Acad. Sci. U. S. A. 111:7024–7029. doi: 10.1073/pnas.1401478111
Watanabe, D., Gotoh, H., Miura, T., and Maekawa, K. (2014). Social interactions affecting caste development through physiological actions in termites. Front. Physiol. 5:127. doi: 10.3389/fphys.2014.00127
Watanabe, D., and Maekawa, K. (2008). Frontal-pore formation during soldier differentiation induced by juvenile hormone III in the termite Reticulitermes speratus (Isoptera: Rhinotermitidae). Sociobiology 52, 437–447.
Watson, J. A. L., and Abbey, H. M. (1985). “Development of neotenics in Mastotermes darwiniensis Froggatt: an alternative strategy,” in Caste Differentiation in Social Insects, eds J. A. L. Watson, B. M. Okot-Kotber, and C. H. Noirot (Oxford: Pergamon Press), 107–124. doi: 10.1016/b978-0-08-030783-1.50013-6
Weesner, F. M. (1969). “External anatomy,” in Biology of termites Vol I, eds K. Krishna and F. M. Weesner (New York: Academic Press) 19–47. doi: 10.1016/b978-0-12-395529-6.50006-4
Wheeler, D. E., and Nijhout, H. F. (1981). Imaginal wing discs in larvae of the soldier caste of Pheidole bicarinata vinelandica Forel (Hymenoptera: Formicidae). Int. J. Insect Morphol. Embryol. 10, 131–139. doi: 10.1016/s0020-7322(81)80018-9
Yang, A. S., and Abouheif, E. (2011). Gynandromorphs as indicators of modularity and evolvability in ants. J. Exp. Zool. B Mol. Dev. Evol. 316, 313–318. doi: 10.1002/jez.b.21407
Keywords: termite, caste differentiation, neotenic, modularity, heterochrony, developmental arrest
Citation: Oguchi K, Maekawa K and Miura T (2021) Regulatory Mechanisms Underlying the Differentiation of Neotenic Reproductives in Termites: Partial Release From Arrested Development. Front. Ecol. Evol. 9:635552. doi: 10.3389/fevo.2021.635552
Received: 30 November 2020; Accepted: 05 February 2021;
Published: 25 February 2021.
Edited by:
Solange Del Carmen Issa Ponce, Simón Bolívar University, VenezuelaReviewed by:
Ives Haifig, Federal University of ABC, BrazilSimon Hellemans, Okinawa Institute of Science and Technology Graduate University, Japan
Copyright © 2021 Oguchi, Maekawa and Miura. This is an open-access article distributed under the terms of the Creative Commons Attribution License (CC BY). The use, distribution or reproduction in other forums is permitted, provided the original author(s) and the copyright owner(s) are credited and that the original publication in this journal is cited, in accordance with accepted academic practice. No use, distribution or reproduction is permitted which does not comply with these terms.
*Correspondence: Kohei Oguchi, k-oguchi@aist.go.jp; k.ohgreen226@gmail.com