Surface Functionalization of Cotton Fabrics by Photo-Grafting for pH Sensing Applications
- 1Department of Applied Science and Technology, Politecnico di Torino, Turin, Italy
- 2Department of Engineering and Applied Sciences, University of Bergamo, Bergamo, Italy
This paper investigates the photo-grafting of a halochromic molecule on cotton fabric surfaces. Nitrazine yellow (NY) dye was modified with a methacrylate moiety (GMA) obtaining a photo-reactive dye (GMA-NY), which was covalently grafted onto the cellulosic substrate by means of UV irradiation. The photo-grafting reaction was studied by FT-IR spectroscopy, while the pH response of the functionalized fabric was evaluated at different pH conditions (i.e., acidic and alkaline conditions) both in wet and vapor environments by spectrophotometric analyses. The results showed that the photo-grafting of the modified dye onto the fabric was successful: treated cotton clearly changed color when subjected to different pH. It was thus demonstrated that the GMA-NY dye, photo-grafted on cotton, can successfully impart pH sensing properties to the fabric, which can therefore find numerous applications in the area of smart textiles.
Introduction
Stimuli-responsive textiles have been rapidly developed over recent decades. A growing interest is attributed toward smart textiles with reversible color change characteristics that are triggered by an external stimulus. Color change can take place due to diverse stimuli, including temperature, light, electricity, and pH (Mather, 2001; Rubacha, 2007; Van der Schueren and De Clerck, 2010; Hu et al., 2012; Van der Schueren and De Clerck, 2012). The color change in halochromic dyes mainly relies on protonation and de-protonation of a dye molecule (i.e., tautomerism), which leads to the modification of its electron configuration. One of the main classes of halochromic dyes are azo-dyes, such as nitrazine yellow (NY), known for being a proton donor species (Pavlović et al., 2009; Van der Schueren and De Clerck, 2012; Van der Schueren et al., 2012b).
Halochromic textiles are potential candidates for sensor devices: the color switch due to a pH change can be immediately visible and can thus act as a signal of the system modification. Textile-based sensor systems are flexible, breathable, easy to handle, and comfortable, compared with conventional sensors; they also have the advantage of large area coverage (Christie, 2013). As a result, these smart textiles have numerous applications in several fields, such as protective clothing, wound healing, filtration, etc. (Wojciechowski, 1996; Osti, 2008; Nanda et al., 2010). For instance, in the medical and diagnostic field, a pH sensor incorporated into a textile fabric could monitor in real-time the biochemical changes in body fluids, such as during sweating: wearable textile-based pH sensors can measure the dehydration level of athletes during exercise by directly monitoring the pH of their sweat (Morris et al., 2009; Caldara et al., 2016).
Among textiles, cotton is preferred as substrate for various functionalizations: cellulose, as a naturally occurring polymer and thanks to its abundance and low cost, is in fact one of the most promising available raw materials. Moreover, it has been shown that cellulosic fabrics are excellent carriers for pH-indicator dyes (Van der Schueren and De Clerck, 2012; Gürdağ and Sarmad, 2013).
Fabric functionalization with halochromic dyes is generally performed by conventional exhaustion dyeing (Khatri et al., 2015), surface treatment and coating (Galbraith, 2004; Cheng et al., 2008), sol-gel process (Miled et al., 2002; Trovato et al., 2018), or microencapsulation technique (Nelson, 2002). However, many of these methods can suffer from long dyeing time at high temperature, insufficient exhaustion and fixation levels, and inadequate affinity of dye and fibers, which result in poor treatment durability, dye leaching and slow sensor response time when the dye is incorporated into the fabric. Therefore, an alternative method to durably functionalize fabrics with dyes and reduce the process time and energy is highly needed. Indeed coupling the dye with a linking agent able to form covalent bonds between the dye molecule and the fabric can minimize dye leaching (Van der Schueren et al., 2012a).
A covalent grafting process is thus preferred and can be applied selectively at the textile surface. Photo-induced grafting is an accessible and eco-friendly method for surface modification (Rånby, 1998). It is a surface treatment method, in which the functional moieties are grafted only onto the outermost layer of the substrate (e.g., approximate depth of 200 μm), without modifying its bulk (Khan, 2004). Photo-grafting has already demonstrated to be a successful technique to modify cellulose-based materials (Hassan and Khan, 2009; Bongiovanni et al., 2011; Bongiovanni et al., 2013; Kang et al., 2015) and in particular cotton fabrics (Hong et al., 2009; Ferrero et al., 2015; Periolatto et al., 2016).
Photo-grafting reactions involve hydrogen abstraction from the fiber carbon chain in the presence of a proper photoinitiator, as a consequence of light energy absorption (generally UV light). The reaction propagates by radical formation and then leads to the covalent bonding of the dye to the fabric surface. This technique has many advantages: (i) many functional monomers can be grafted to the substrate, (ii) the reaction is fast and controllable, (iii) it occurs at room temperature and thus can be applied to thermo-sensitive substrates, and (iv) more importantly it guarantees long grafting durability (Dong and Jang, 2014).
Thanks to its interesting application in several biological and diagnostic fields, NY as a halochromic molecule, has been used in the development of smart textiles. In a previous investigation (Guido et al., 2014), NY was immobilized onto cotton fabrics by the sol-gel technique, with the aim of developing wearable pH-meter textile. The authors demonstrated the covalent functionalization of the NY molecules using 3-glycidoxypropyltrimethoxysilane precursor (GPTMS) through the catalyzed epoxy ring-opening by BF3OEt2. The so obtained silica functionalized halochromic dyestuff showed pH-sensitive properties even after its immobilization onto cotton fabrics. Both the GPTMS-NY solution and finished cotton samples gave their color change from yellow (at 466 nm) in acidic conditions to blue (590 nm) in alkaline conditions, showing the same behavior of the pure NY. Compared to the solution, the collected visible reflectance spectra of GPTMS-NY finished cotton samples evidenced a redshift of both wavelengths due to a variation of the microenvironment.
In this work, in order to prepare pH-sensitive cotton fabrics, a halochromic dye functionalized with a methacrylate end group (GMA-NY) was photo-grafted onto the cellulosic surface in the presence of a photoinitiator. The photo-induced process was employed as a more environmentally friendly alternative to the use of the BF3OEt2 catalyst. The chemical structure of both the dye and the cotton substrate, as well as the grafting efficiency were assessed by Attenuated Total Reflectance Fourier Transform Infrared (ATR FT-IR) spectroscopy, and the halochromic response of the treated cotton was demonstrated by spectrophotometric measurements.
Materials and Methods
Materials
The NY dye modified by a glycidyl methacrylate group (GMA-NY) was synthesized by following an optimized sol-gel reaction described in previous works (Guido et al., 2014; Caldara et al., 2016). Briefly, the glycidyl methacrylate (GMA) molecule was linked to the NY dye by ring opening of GMA in acidic conditions (water solution of the Lewis acid boron trifluoride diethyl etherate BF3OEt2). The obtained modified dye was then diluted to 0.06 M aqueous solution. The molecular structure of GMA-NY, together with its halochromic protonation and de-protonation mechanism, is reported in Figure 1.
The cotton fabric (100% plain-weave, scoured and bleached, mass per unit area of 331 g/m2) was kindly provided by Mascioni S.p.A. (Cuvio, Italy). Prior to use, the fabric was washed (2% non-ionic detergent, pH 7 and 40°C for 20 min), rinsed with deionized water, dried, and equilibrated at standard atmospheric conditions for at least 24 h.
Benzophenone (≥99%), ethanol (≥99.8%) and all other chemicals were purchased from Sigma-Aldrich and used as received.
Photo-Grafting
Benzophenone photoinitiator (2 wt % with respect to the weight of the dye) was added to the 0.06 M diluted solution of GMA-NY. The cotton fabric was cut into 8 cm × 8 cm samples and dipped in the dye solution for a given period of time (10–60 s). After removing the excess of liquid by a soft tissue paper, the impregnated samples were subjected to UV irradiation by a metal halide lamp (Dymax ECE 5000 flood lamp), with a UV intensity of 110 mW/cm2 for 2 min on each side of the samples. The photo-grafting was carried out under inert atmosphere by constant nitrogen flow. After irradiation, rinsing was performed in two cycles to remove the dye that was not chemically grafted to the fabric (e.g., unreacted species and GMA-NY homopolymer): samples were immersed for 24 h in distilled water, and the water bath was changed after the first 2 h of sample immersion. Afterward, rinsed samples were dried in oven for 30 min at 70°C prior to characterization.
Characterization
In order to measure the halochromic properties and viability of both pristine (non-irradiated) and UV-irradiated GMA-NY solution, UV–vis measurements were performed. Absorbance spectra were collected by means of a 6850 Jenway UV–vis spectrophotometer in the range of 300-800 nm with a resolution of 0.5 nm, by using cuvettes with path length of 1 cm. The dye solution was diluted 1:1000 and then investigated, both before and after light irradiation.
The dye activity in acidic and alkaline conditions was also assessed by UV–vis spectroscopy analyses. The UV-irradiated GMA-NY solution was introduced to four different aqueous solutions having a pH between 2 and 10 (i.e., pH 2.5, 6, 7, and 9.5). The pH buffer solutions were prepared using HCl and NaOH.
The dye wet pick-up of the cotton samples for different impregnation times was measured by gravimetric method, based on the weight change of the fabrics according to Eq. 1:
where Wi is the initial weight of the sample prior to impregnation, and Wf is its final weight after impregnation. For each sample at least three measurements were repeated, the obtained values were averaged, and the standard deviation was calculated. Measurements were conducted in standard laboratory conditions: 23°C, 45–49% relative humidity.
For assessing the photo-grafting efficiency of the halochromic system onto the cotton fabric, the chemical bonds and composition of the samples prior and after irradiation were investigated by ATR FT-IR spectroscopy analyses, using a NicoletTM iS50 FTIR spectrometer (Thermo ScientificTM) with a diamond crystal. A resolution of 4 cm–1 and 32 scans per sample were applied.
The pH response of treated fabrics was investigated through spectrophotometric (data-color) analysis, using a Datacolor Check II Plus spectrophotometer in reflectance mode under the condition of D65 illuminant and 10° standard observer. Fabric samples were introduced in acidic and alkaline aqueous solutions (prepared using HCl and NaOH, respectively) for 2 min and the color change was measured immediately on wet samples. The color change of the samples was also evaluated after their exposure in a closed chamber to acidic and alkaline vapors for 24 h. In both cases (i.e., wet and vapor conditions), the acid pH corresponded to ≈3.5, while the alkali pH corresponded to ≈8.5. For each sample at least three measurements were repeated: the obtained values were averaged and the error was calculated. A plain untreated cotton fabric was used as reference sample. UV-grafted cotton fabric, prior to the exposure to different pH conditions, was instead used as control sample.
The CIE (International Commission of Illumination) LAB values were analyzed to assess the color changes of the fabrics. The total color difference is represented by ΔE, obtained from Eq. 2:
where L, a, and b are color space coordinates, specifically lightness, green-red and yellow-blue coordinates, respectively. In particular, negative and positive values of a represent the position in green and red color regions, similarly negative and positive values of b are associated to blue and yellow color regions, respectively. Generally, samples with CIE ΔE value from 2 to 4 have perceivable color difference, those between 4 and 5 have significant color difference, and above 12 belong to a different color space (Wyszecki and Fielder, 1971; Jafari-Kiyan et al., 2017). In this work, in addition to ΔE, the value of Δb was also monitored due to the characteristic color changes of the GMA-NY system, mainly taking place in the yellow-blue color region.
The reflectance values acquired by the spectrophotometric analyses were employed to calculate the corresponding Kubelka–Munk function. Kubelka–Munk theory (Kubelka and Munk, 1931) is a simplified analysis of the interaction of light and a layer of material, which can predict the light scattering properties including reflectance (R), transmittance, absorption, etc. Kubelka and Munk explained the light propagation by dividing the thickness of a sample into a series of layers with equal thickness parallel to an opaque substrate. When the light flux is traveling through the layers, according to Beer-Lambert law, its irradiance changes, and these changes depend on two constants called absorption coefficient (K) and scattering coefficient (S). Kubelka–Munk theory presents an analytical expression for the reflectance R of the sample in terms of absorption and scattering coefficients. In particular, the ratio of the two parameters K and S can be obtained by Eq. 3:
where the reflectance R is substituted with R∞ (R = R∞), as the textile is considered an opaque material (Kubelka and Munk, 1931; El Sherif et al., 1997; Berns and Mohammadi, 2007; Džimbeg-Malčić et al., 2011; Sadeghi-Kiakhani and Safapour, 2015).
Results and Discussion
Photo-Grafting Process
The photo-grafting mechanism of the modified halochromic GMA-NY dye onto the cellulosic material can be described as shown in Figure 2A. The photo-induced reaction is triggered by UV light: ground state molecules of photoinitiator absorb photons, which can have sufficient energy to produce triplet state excited species (obtained through intersystem crossing from the first singlet state). These triplet state species of the photoinitiator abstract hydrogen from the substrate RH, forming radicals. In particular, in the presence of a cotton substrate, the photoinitiator abstracts hydrogen atoms from the abundant hydroxyl groups of cellulose, thus generating cellulose radicals (Roy et al., 2009; Chen et al., 2016). Such radicals can attack the carbon double bonds of the methacrylate groups of GMA-NY, covalently bonding the dye and the cellulose, and initiating chain propagation reactions. Therefore, under UV irradiation and in the presence of a photoinitiator, GMA-NY graft chains can be grown from the cotton substrate (Figure 2B). Simultaneously, the competing reaction of GMA-NY homopolymerization in solution (i.e., not grafted to the solid substrate) can occur. It is also expected that the chromophore group in the dye molecule plays a role in exciting the GMA moiety of the dye, further enhancing the radical reaction.
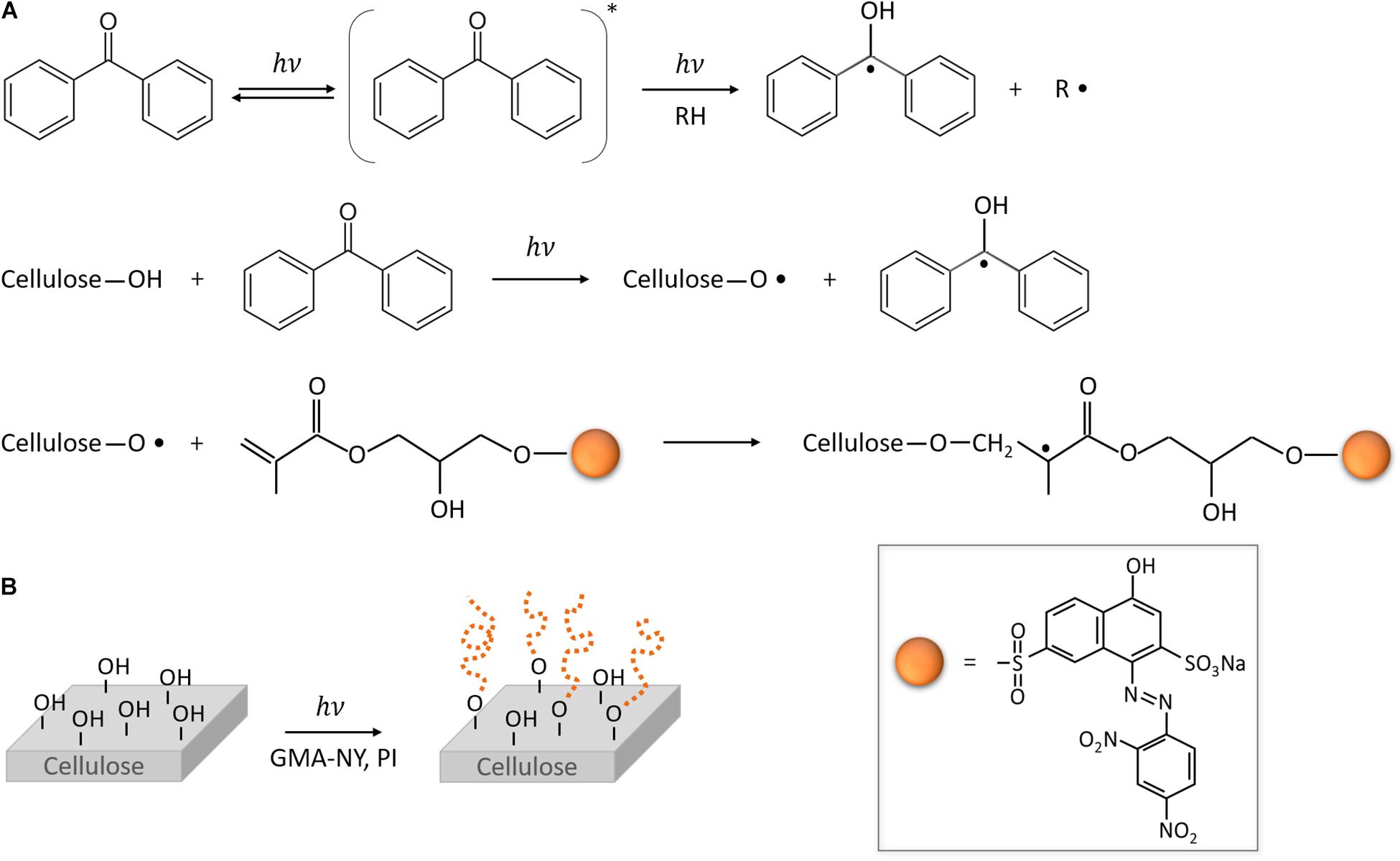
Figure 2. Photo-grafting of GMA-NY onto cotton. (A) Mechanism of the photo-grafting reaction of GMA-NY onto cotton (cellulose-based material), in the presence of benzophenone as photoinitiator. ∗ indicates the excited state. (B) Scheme of the photo-grafting process of cotton substrate by light irradiation, in the presence of the functionalized GMA-NY dye and the photoinitiator (PI).
Prior to photo-grafting of GMA-NY system on the textile fabric, the proper impregnation time of cotton samples was optimized. The cotton fabric samples were immersed into the dye solution for different time periods between 10 and 60 s and the GMA-NY wet pick-up was measured by weight. Results are reported in Figure 3: as expected, the impregnation of the samples is very fast and reaches a plateau after few seconds. The impregnation time of 30 s, giving a wet pick-up of around 85%, was selected to proceed with the irradiation of the fabric.
After impregnation with the solution containing GMA-NY and the photoinitiator, the cotton samples were subjected to UV irradiation, washing and drying. The washing procedure was performed as described in the Experimental Section to remove unreacted species, chains of GMA-NY homopolymer and any molecule that was not covalently linked to the fabric. As will be discussed in detail below, the photo-grafting process led to a slight color change of the fabric sample surface (visually inspected).
To investigate the occurrence of the photo-grafting of GMA-NY onto the cotton fabric, ATR FT-IR spectroscopy analysis of treated samples was performed and compared with that of untreated cotton and GMA-NY dye solution (Figure 4).
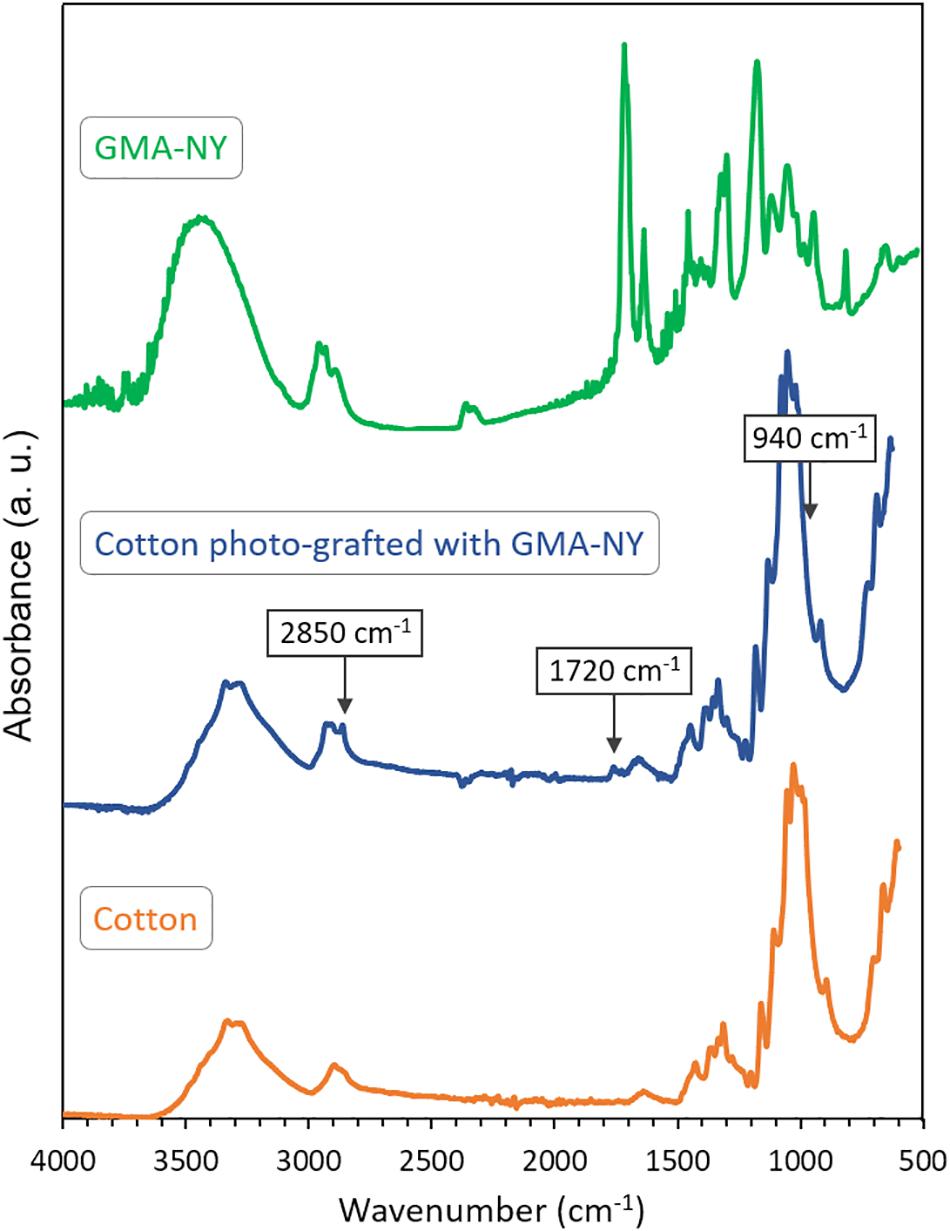
Figure 4. ATR FT-IR spectra of GMA-NY, untreated cotton fabric and cotton fabric photo-grafted with GMA-NY.
In both untreated and treated cotton samples, the main characteristic peaks of cellulose are present at 3500–3100 cm–1 (O–H stretching), 3000–2800 cm–1 (C–H asymmetric stretching vibrations), 1427–1201 cm–1 (O–H in-plane bending, C–H bending, –CH2 rocking, C–H deformation stretching and C–O stretching), and 1159–895 cm–1 (C–O–C asymmetric bridge stretching, asymmetric in-plane and out-of-phase ring stretching, C–O ring stretching). Moreover the peak at 1640 cm–1 can be attributed to bending mode of O–H of absorbed water (Chung et al., 2004; Abidi et al., 2010; Abidi et al., 2014).
In the FT-IR spectrum of the GMA-NY solution, peaks at 3000–2800, 1716, 1637, and 940 cm–1 are assigned to C–H asymmetric stretching vibrations (in GMA-NY structure), ester carbonyl stretching (in acrylic moiety of GMA), stretching of C–C conjugated double bond (in acrylic moiety of GMA), and C=C wagging (in acrylic moiety of GMA), respectively (Deniau et al., 2006; Rosace and Massafra, 2008; Xu et al., 2012; Chiappone et al., 2014).
In order to confirm the grafting of GMA-NY monomers onto cotton samples, the FT-IR spectrum of cotton fabric grafted with GMA-NY (after thoroughly rinsing) was compared with those of the reference textile and the functionalized dye (Figure 4). In general, the spectrum of photo-grafted cotton demonstrates a slight shift of all peaks of few wavenumbers, compared to the spectrum of untreated cotton. It is important to notice that the peaks attributed to the dye molecule are present, but the functionalization is not clearly characterizable since the main infrared peaks are covered by the strong vibrational peaks of the cellulosic substrate.
However, as shown in Figure 4, the peak band at around 1720 cm–1 (C=O stretching) can be recognized as characteristic peak of the dye molecule grafted on cotton. In fact, as the photo-grafting process was carried out under continuous nitrogen flux, oxidation of cellulose could be excluded. The absence of C=C wagging at 942 cm–1 confirms the successful grafting of GMA-NY onto cotton fabric. Moreover, the increase of intensity observed in the peak at 2851 cm–1 may be due to a combined effect of C–H stretching vibration of methyl group of GMA-NY and C–H stretching of cellulose.
pH Response of the Photo-Grafted Substrate
GMA-NY was selected in this work as it can be covalently linked to cellulose by photo-grafting thanks to its methacrylate moiety and thus can impart pH sensing properties to the cotton fabric. Preliminary tests were conducted to confirm that the pH response of GMA-NY dye was not influenced by UV irradiation. UV–vis spectroscopy of the dye solution was performed before and after its exposure to UV light with the above-mentioned curing conditions (i.e., 2 min, 110 mW/cm2 under nitrogen atmosphere).
Figure 5 shows the UV–vis spectra of GMA-NY solution recorded before and after UV irradiation (Figure 5A) and the halochromic behavior of UV-irradiated GMA-NY at different pH values (Figures 5B,C).
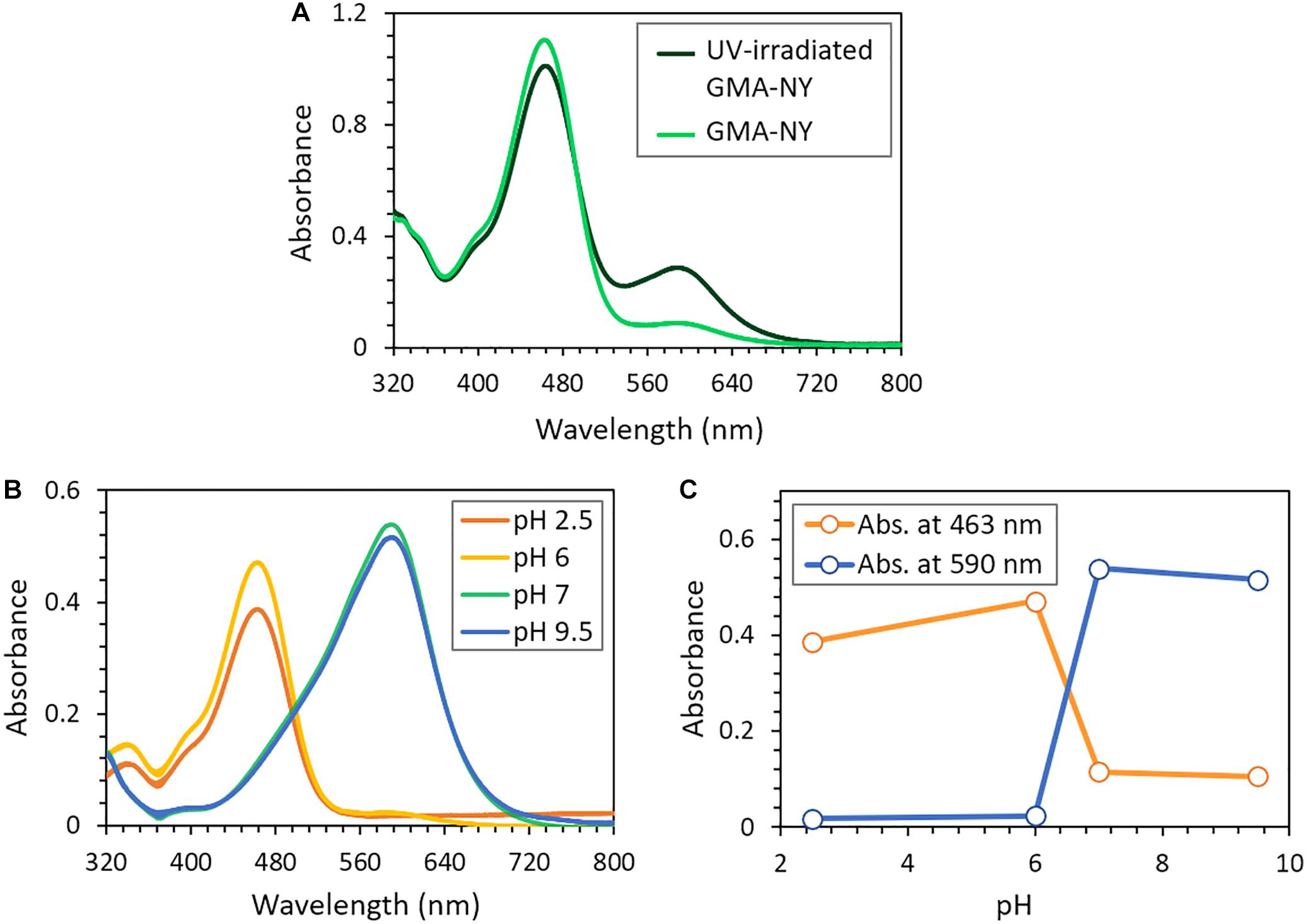
Figure 5. Halochromic response of GMA-NY solution after UV irradiation. (A) UV–vis spectra of the dye solution before and after exposure to UV light. (B) UV–vis spectra of the UV-irradiated dye solution at different pH values. (C) UV–vis absorbance values at 463 nm and 590 nm of the UV-irradiated dye solution as a function of pH.
As shown in Figure 5A, the GMA-NY spectrum is slightly changed after irradiation, although the dye degradation by UV light can be excluded since the visible bands do not shift or disappear. Moreover, it is worth noticing that the dye absorption peak is centered around 463 nm, and hence it cannot affect the UV light absorption process of the photoinitiator, which occurs at wavelengths lower than 300 nm. In this region, the photo-grafting process acts onto the C=C bonds of the functionalized NY molecule, modifying the immediate chromophore surroundings of the dyestuff. As a consequence, an increase of the band centered at about 590 nm can be observed in the UV–vis spectrum (Figure 5A). Some authors (Beatrup et al., 2014) attributed this phenomenon to the reduction in conjugation length. Accordingly, the reduction of the intensity of the observed band at around 463 nm is probably due to the degradation of the C=C double bond as a consequence of the photo-curing process initiated by benzophenone, as suggested by the FT-IR evidence described above.
Subsequently, buffer solutions of different pH ranging between 2 and 10 were added to the UV-irradiated GMA-NY solution: a clear color change from yellow to blue, distinctly visible by naked eye, was observed, in accordance with NY halochromic properties (Guido et al., 2014). UV–vis spectroscopy was also used to better monitor the dye activity. Figure 5B shows that the spectrum of the UV-irradiated dye has absorbance maximum peaks at 463 nm and 590 nm in acidic and alkaline conditions, respectively. Figure 5C represents the maximum dye absorbance at these two wavelengths as a function of pH. It is observed that GMA-NY halochromic response to acidic and alkaline conditions takes place mainly in the range of pH between 6 and 7. It is also demonstrated that the dye activity is preserved after UV irradiation at all pH conditions.
A quantitative characterization of the halochromic behavior of cotton samples functionalized with GMA-NY was performed by measuring their color change through spectrophotometric analysis. The Kubelka–Munk function was used to convert the diffuse reflectance values for different wavelengths into the K/S ratio (Figure 6A), which is a measure of the fabric color strength. A plain untreated cotton fabric, which shows a similar trend as white color in the reflectance spectrum, was used as standard sample. UV-grafted cotton fabric, prior to the exposure to different pH conditions, was instead used as control sample. In Figure 6A, the variation of the K/S spectrum of the control from the standard sample is evident, as the treated cotton has a pale yellowish color (also shown in Figure 6B).
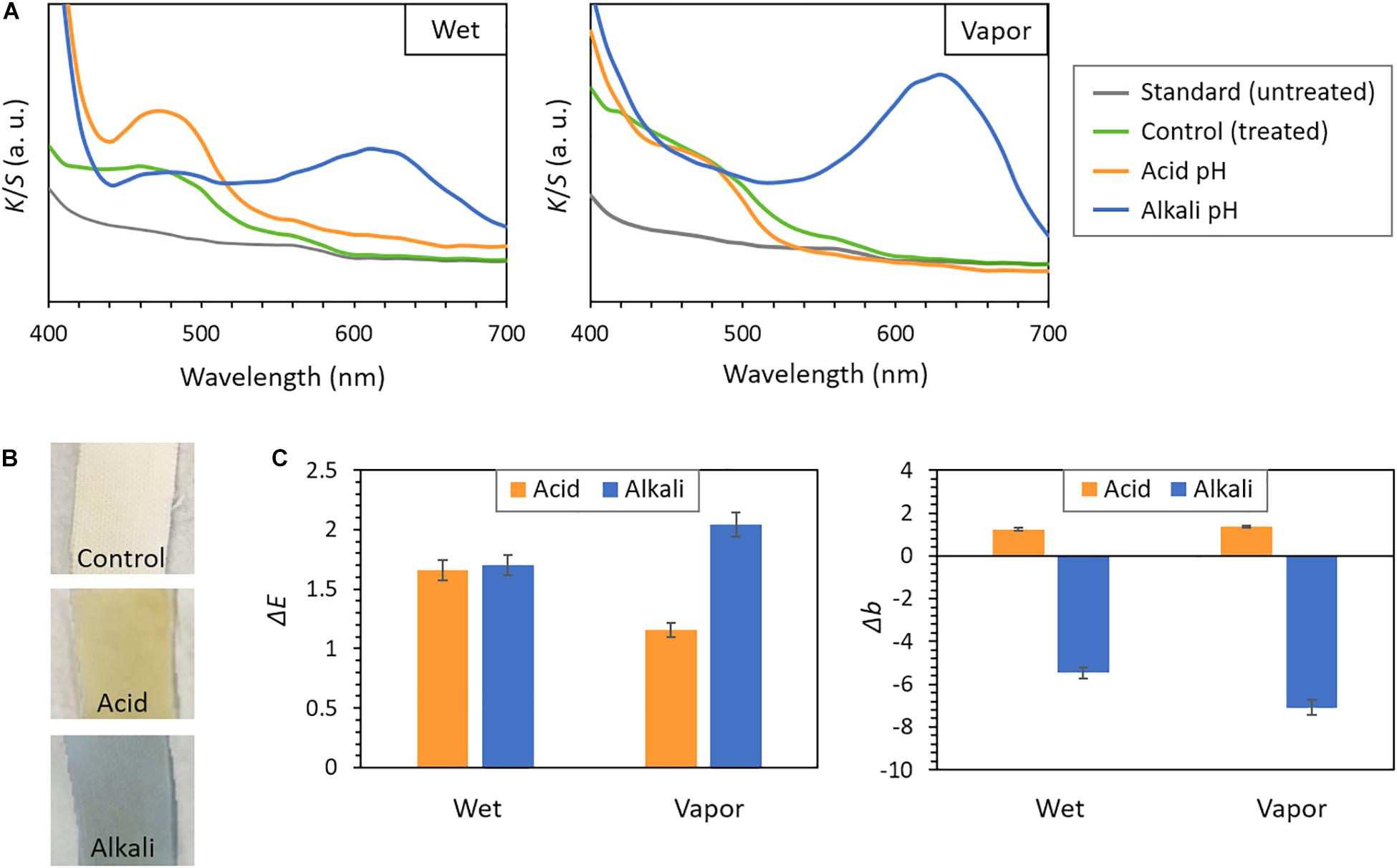
Figure 6. pH response of cotton fabrics photo-grafted with GMA-NY. (A) K/S versus wavelength spectra, obtained by the Kubelka–Munk function, for samples subjected to acidic and alkaline pH in both wet and vapor conditions. (B) Images of the photo-grafted cotton fabric samples, after storage in atmospheric conditions (control), and after immersion in acidic and alkaline pH buffer solutions. (C) ΔE and Δb values of samples subjected to acidic and alkaline pH in both wet and vapor conditions, with respect to a photo-grafted cotton fabric stored in atmosphere air (i.e., control sample). Data were obtained by spectrophotometric analysis.
Cotton fabrics photo-grafted with GMA-NY were subjected to acidic and alkaline conditions (pH of ≈3.5 and 8.5, respectively) as described in the Experimental Section. In particular, the assessment in wet conditions was performed immediately after immersion of the samples for 2 min in different pH buffer solutions, while the measurement for vapor condition was performed after 24 h of exposure to acid or alkali vapors. All grafted samples present a clearly visible color change from yellow in acidic environment to blue in basic environment (Figure 6B), thus demonstrating that the functionalized fabrics exhibit an obvious and fast pH response. Accordingly, as shown in Figure 6A, the treated samples present different K/S versus wavelength spectra under various pH conditions.
A bathochromic shift of maximum peaks, compared to the corresponding dye solution, could be detected: the acidic maximum shifted from 463 nm in the dye solution to 470 nm on the fabric, while the alkaline maximum shifted from 590 nm to 630 nm. Acidic and alkaline maxima intensities of Kubelka–Munk curves are clearly affected by the pH, confirming the effectiveness of the halochromic response of cotton fabrics photo-grafted with GMA-NY. In particular, the acidic pH conditions lead to typical K/S spectra of yellow color; whereas, the alkaline pH conditions result in the typical spectra of blue color. Although similar trends are obtained for the results in wet and vapor conditions, the response to acidic pH seems to be more pronounced in wet conditions, while the response to alkaline pH in vapor conditions.
The pH-sensitive color change of the cotton samples photo-grafted with GMA-NY was also analytically assessed by analyzing the ΔE values of data-color spectrophotometer. The halochromic response of treated cotton to different pH environments (both in wet and vapor conditions) was examined with respect to a photo-grafted cotton fabric stored in atmosphere air (i.e., control sample): ΔE results are reported in Figure 6C. When the cotton is subjected to acidic and alkaline condition, an evident color change is not only perceivable by naked eye (Figure 6B), but can also be analytically detected (Figure 6C). The shift in ΔE value, compared to GMA-NY-treated cotton, is similar for both aqueous and vapor conditions.
However, the measured values of ΔE do not allow to distinguish between a treated cotton subjected to an acidic or an alkaline environment, especially for wet conditions. Therefore, the Δb values in CIELAB of the samples, which describe the color changes from blue (negative) to yellow (positive), were also analyzed by spectrophotometric measurements. The measured Δb values with respect to the control photo-grafted cotton fabric stored in atmosphere air (Figure 6C) show an obvious change from acidic to alkaline pH conditions for all samples subjected to pH buffer solutions and vapors. In particular, the blue color of the treated samples in alkaline environments is evidently detected (Δb < −5).
Conclusion
In this study we investigated the grafting of a modified NY dye with a methacrylate group (GMA-NY) on cotton fabric by photo-induced reaction. The photo-grafting of the halochromic agent was performed by UV irradiation in the presence of benzophenone as photoinitiator and under inert atmosphere. The fabric dipping time into the dye solution was optimized by evaluating its wet pick-up. The photo-grafting reaction, which consists in the covalent bonding of the dye to the cellulosic backbone of cotton, was investigated by FT-IR spectroscopy. The halochromic and pH response of treated cotton was analyzed by CIELAB color changes and UV–vis reflectance measurements. When the fabric was subjected to acidic and alkaline environments (both in wet and vapor conditions), a color change occurred, which was both clearly visible by naked eye and significantly detected by analytical instruments. It was thus demonstrated that the modified GMA-NY dye, photo-grafted on cotton surfaces, can successfully impart pH sensing properties to the fabric, paving the way for a number of applications of the proposed process in the area of smart textiles. Further investigation will be performed in terms of on-body trials to test the pH-sensor performance of the UV-grafted GMA-NY cotton textiles for potential applications in sport, fitness, wellness, healthcare, and medicine fields.
Data Availability Statement
The datasets generated for this study are available on request to the corresponding author.
Author Contributions
GR, AF, RB, and AV conceived and designed the experimental work. PK, MA, VT, and AV carried out the experiments. All authors contributed to writing the manuscript.
Conflict of Interest
The authors declare that the research was conducted in the absence of any commercial or financial relationships that could be construed as a potential conflict of interest.
References
Abidi, N., Cabrales, L., and Haigler, C. H. (2014). Changes in the cell wall and cellulose content of developing cotton fibers investigated by FTIR spectroscopy. Carbohydr. Polym. 100, 9–16. doi: 10.1016/j.carbpol.2013.01.074
Abidi, N., Cabrales, L., and Hequet, E. (2010). Fourier transform infrared spectroscopic approach to the study of the secondary cell wall development in cotton fiber. Cellulose 17, 309–320. doi: 10.1007/s10570-009-9366-1
Beatrup, D., Wade, J., Biniek, L., Bronstein, H., Hurhangee, M., Kim, J.-S., et al. (2014). Polaron stability in semiconducting polymer neat films. Chem. Commun. 50, 14425–14428. doi: 10.1039/c4cc06193d
Berns, R. S., and Mohammadi, M. (2007). Single-constant simplification of Kubelka-Munk turbid-media theory for paint systems—a review. Color Res. Appl. 32, 201–207. doi: 10.1002/col.20309
Bongiovanni, R., Marchi, S., Zeno, E., Pollicino, A., and Thomas, R. (2013). Water resistance improvement of filter paper by a UV-grafting modification with a fluoromonomer. Colloids Surf. A Physicochem. Eng. Asp. 418, 52–59. doi: 10.1016/j.colsurfa.2012.11.003
Bongiovanni, R., Zeno, E., Pollicino, A., Serafini, P., and Tonelli, C. (2011). UV light-induced grafting of fluorinated monomer onto cellulose sheets. Cellulose 18, 117–126. doi: 10.1007/s10570-010-9451-5
Caldara, M., Colleoni, C., Guido, E., Re, V., and Rosace, G. (2016). Optical monitoring of sweat pH by a textile fabric wearable sensor based on covalently bonded litmus-3-glycidoxypropyltrimethoxysilane coating. Sens. Actuators B Chem. 222, 213–220. doi: 10.1016/j.snb.2015.08.073
Chen, M., Zhong, M., and Johnson, J. A. (2016). Light-controlled radical polymerization: mechanisms, methods, and applications. Chem. Rev. 116, 10167–10211. doi: 10.1021/acs.chemrev.5b00671
Cheng, T., Lin, T., Brady, R., and Wang, X. (2008). Fast response photochromic textiles from hybrid silica surface coating. Fibers Polym. 9, 301–306. doi: 10.1007/s12221-008-0048-7
Chiappone, A., Nair, J., Gerbaldi, C., Zeno, E., and Bongiovanni, R. (2014). Flexible and high performing polymer electrolytes obtained by UV-induced polymer–cellulose grafting. RSC Adv. 4, 40873–40881. doi: 10.1039/c4ra07299e
Christie, R. (2013). “Chromic materials for technical textile applications,” in Advances in the Dyeing and Finishing of Technical Textiles, ed. M. L. Gulrajani (Oxford: Woodhead Publishing), 3–36. doi: 10.1533/9780857097613.1.3
Chung, C., Lee, M., and Choe, E. K. (2004). Characterization of cotton fabric scouring by FT-IR ATR spectroscopy. Carbohydr. Polym. 58, 417–420. doi: 10.1016/j.carbpol.2004.08.005
Deniau, G., Charlier, J., Alvado, B., Palacin, S., Aplincourt, P., and Bauvais, C. (2006). Study of the simultaneous electro-initiated anionic polymerization of vinylic molecules. J. Electroanal. Chem. 586, 62–71. doi: 10.1016/j.jelechem.2005.09.019
Dong, Y., and Jang, J. (2014). Environmentally friendly coloration of cotton by the UV-induced photografting of α-bromoacrylamido dyes. Coloration Technol. 130, 296–304. doi: 10.1111/cote.12094
Džimbeg-Malčić, V., Barbarić-Mikočević, Ž, and Itrić, K. (2011). Kubelka-Munk theory in describing optical properties of paper (I). Tehnički Vjesnik 18, 117–124. doi: 10.1016/j.aca.2008.03.063
El Sherif, M., Bayoumi, O., and Sokkar, T. (1997). Prediction of absorbance from reflectance for an absorbing-scattering fabric. Color Res. Appl. 22, 32–39. doi: 10.1002/(sici)1520-6378(199702)22:1<32::aid-col6>3.0.co;2-5
Ferrero, F., Periolatto, M., and Ferrario, S. (2015). Sustainable antimicrobial finishing of cotton fabrics by chitosan UV-grafting: from laboratory experiments to semi industrial scale-up. J. Clean. Prod. 96, 244–252. doi: 10.1016/j.jclepro.2013.12.044
Galbraith, J. (2004). An assessment of the technical performance of photochromic dyes in textile printing. AATCC Rev. 4, 26–30.
Guido, E., Colleoni, C., De Clerck, K., Plutino, M. R., and Rosace, G. (2014). Influence of catalyst in the synthesis of a cellulose-based sensor: kinetic study of 3-glycidoxypropyltrimethoxysilane epoxy ring opening by Lewis acid. Sens. Actuators B Chem. 203, 213–222. doi: 10.1016/j.snb.2014.06.126
Gürdağ, G., and Sarmad, S. (2013). “Cellulose graft copolymers: synthesis, properties, and applications,” in eds Polysaccharide Based Graft Copolymers, S. Kalia, M. Sabaa (Berlin: Springer), 15–57. doi: 10.1007/978-3-642-36566-9_2
Hassan, M. M., and Khan, M. A. (2009). Effect of radiation pretreatment on photo-grafting of acrylamide on cellulose. J. Adh. Sci. Technol. 23, 247–257. doi: 10.1163/156856108x369598
Hong, K. H., Liu, N., and Sun, G. (2009). UV-induced graft polymerization of acrylamide on cellulose by using immobilized benzophenone as a photo-initiator. Eur. Polym. J. 45, 2443–2449. doi: 10.1016/j.eurpolymj.2009.04.026
Hu, J., Meng, H., Li, G., and Ibekwe, S. I. (2012). A review of stimuli-responsive polymers for smart textile applications. Smart Mater. Struct. 21:053001. doi: 10.1088/0964-1726/21/5/053001
Jafari-Kiyan, A., Karimi, L., and Davodiroknabadi, A. (2017). Producing colored cotton fabrics with functional properties by combining silver nanoparticles with nano titanium dioxide. Cellulose 24, 3083–3094. doi: 10.1007/s10570-017-1308-8
Kang, H., Liu, R., and Huang, Y. (2015). Graft modification of cellulose: methods, properties and applications. Polymer 70, A1–A16. doi: 10.1016/j.colsurfb.2012.10.025
Khan, F. (2004). Photoinduced graft—copolymer synthesis and characterization of methacrylic acid onto natural biodegradable lignocellulose fiber. Biomacromolecules 5, 1078–1088. doi: 10.1021/bm049967b
Khatri, A., Peerzada, M. H., Mohsin, M., and White, M. (2015). A review on developments in dyeing cotton fabrics with reactive dyes for reducing effluent pollution. J. Clean. Produc. 87, 50–57. doi: 10.1016/j.jclepro.2014.09.017
Miled, O. B., Ouada, H. B., and Livage, J. (2002). pH sensor based on a detection sol–gel layer onto optical fiber. Mater. Sci. Eng. C 21, 183–188. doi: 10.1016/s0928-4931(02)00100-5
Morris, D., Coyle, S., Wu, Y., Lau, K. T., Wallace, G., and Diamond, D. (2009). Bio-sensing textile based patch with integrated optical detection system for sweat monitoring. Sens. Actuators B Chem. 139, 231–236. doi: 10.1016/j.snb.2009.02.032
Nanda, D., Tung, K.-L., Li, Y.-L., Lin, N.-J., and Chuang, C.-J. (2010). Effect of pH on membrane morphology, fouling potential, and filtration performance of nanofiltration membrane for water softening. J. Membr. Sci. 349, 411–420. doi: 10.1016/j.memsci.2009.12.004
Nelson, G. (2002). Application of microencapsulation in textiles. Int. J. Pharm. 242, 55–62. doi: 10.1016/s0378-5173(02)00141-2
Osti, E. (2008). Skin pH variations from the acute phase to re-epithelialization in burn patients treated with new materials (burnshield®, semipermeable adhesive film, dermasilk®, and hyalomatrix®). Non-invasive preliminary experimental clinical trial. Ann. Burns Fire Disasters 21, 73–77.
Pavlović, G., Racane, L., Čičak, H., and Tralić-Kulenović, V. (2009). The synthesis and structural study of two benzothiazolyl azo dyes: X-ray crystallographic and computational study of azo–hydrazone tautomerism. Dyes Pigm. 83, 354–362. doi: 10.1016/j.dyepig.2009.06.002
Periolatto, M., Basit, A., Ferri, A., and Bongiovanni, R. (2016). Wettability and comfort of cellulosic materials modified by photo grafting of non-fluorinated oligomers. Cellulose 23, 1447–1458. doi: 10.1007/s10570-016-0863-8
Rånby, B. (1998). Photoinitiated modifications of polymers: photocrosslinking, surface photografting and photolamination. Mater. Res. Innov. 2, 64–71. doi: 10.1007/s100190050064
Rosace, G., and Massafra, M. R. (2008). Marking of cellulose yarn by vinyl monomer grafting. Text. Res. J. 78, 28–36. doi: 10.1177/0040517507082187
Roy, D., Semsarilar, M., Guthrie, J. T., and Perrier, S. (2009). Cellulose modification by polymer grafting: a review. Chem. Soc. Rev. 38, 2046–2064. doi: 10.1039/b808639g
Rubacha, M. (2007). Thermochromic cellulose fibers. Polym. Adv. Technol. 18, 323–328. doi: 10.1002/pat.889
Sadeghi-Kiakhani, M., and Safapour, S. (2015). Eco-friendly dyeing of treated wool fabrics with reactive dyes using chitosanpoly (propylene imine) dendreimer hybrid. Clean Technol. Environ. Policy 17, 1019–1027. doi: 10.1007/s10098-014-0855-z
Trovato, V., Colleoni, C., Castellano, A., and Plutino, M. R. (2018). The key role of 3-glycidoxypropyltrimethoxysilane sol–gel precursor in the development of wearable sensors for health monitoring. J. Sol Gel Sci. Technol. 87, 27–40. doi: 10.1007/s10971-018-4695-x
Van der Schueren, L., and De Clerck, K. (2010). The use of pH-indicator dyes for pH-sensitive textile materials. Text. Res. J. 80, 590–603. doi: 10.1177/0040517509346443
Van der Schueren, L., and De Clerck, K. (2012). Coloration and application of pH-sensitive dyes on textile materials. Coloration Technol. 128, 82–90. doi: 10.1021/acsnano.7b05139
Van der Schueren, L., De Clerck, K., Brancatelli, G., Rosace, G., Van Damme, E., and De Vos, W. (2012a). Novel cellulose and polyamide halochromic textile sensors based on the encapsulation of Methyl Red into a sol–gel matrix. Sens. Actuators B Chem. 162, 27–34. doi: 10.1016/j.snb.2011.11.077
Van der Schueren, L., Hemelsoet, K., Van Speybroeck, V., and De Clerck, K. (2012b). The influence of a polyamide matrix on the halochromic behaviour of the pH-sensitive azo dye nitrazine yellow. Dyes Pigm. 94, 443–451. doi: 10.1016/j.dyepig.2012.02.013
Wojciechowski, K. (1996). Halochromic effects of azophthalimide dyes and their prediction by PPP method. Dyes Pigm. 32, 199–208. doi: 10.1016/s0143-7208(96)00029-0
Keywords: photo-grafting, pH sensing, cotton functionalization, halochromic dye, smart textiles, wearable sensors
Citation: Kianfar P, Abate MT, Trovato V, Rosace G, Ferri A, Bongiovanni R and Vitale A (2020) Surface Functionalization of Cotton Fabrics by Photo-Grafting for pH Sensing Applications. Front. Mater. 7:39. doi: 10.3389/fmats.2020.00039
Received: 01 August 2019; Accepted: 04 February 2020;
Published: 21 February 2020.
Edited by:
Sher Bahadar Khan, King Abdulaziz University, Saudi ArabiaReviewed by:
Massimiliano D’Arienzo, University of Milano-Bicocca, ItalyEsraa M. Bakhsh, King Abdulaziz University, Saudi Arabia
Copyright © 2020 Kianfar, Abate, Trovato, Rosace, Ferri, Bongiovanni and Vitale. This is an open-access article distributed under the terms of the Creative Commons Attribution License (CC BY). The use, distribution or reproduction in other forums is permitted, provided the original author(s) and the copyright owner(s) are credited and that the original publication in this journal is cited, in accordance with accepted academic practice. No use, distribution or reproduction is permitted which does not comply with these terms.
*Correspondence: Alessandra Vitale, alessandra.vitale@polito.it