Abstract
Fiber lasers, which consist of ideal waveguides of optical fibers, work as stable, practical, and maintenance-free lasers. A passively mode-locked ultrashort pulse fiber laser using the intensity-dependent absorption element has made great progress using a new type of saturable absorber with a nanocarbon material. The techniques of ultrashort pulse amplification and pulse compression were also developed. Using a combination of specialty fibers, ultrawideband pulse sources, such as wavelength tunable ultrashort pulses and supercontinuum, can be demonstrated. These new light sources are useful for laser applications, especially for optical metrology. In this paper, the progress of highly functional ultrashort pulse fiber laser sources and their applications are reviewed mainly on the basis of the works of this author.
Export citation and abstract BibTeX RIS
1. Introduction
An optical fiber is an ultra-low-loss, ideal waveguide. Thus, fiber lasers that consist of optical fibers show excellent performance. Recently, fiber lasers have been recognized as compact, stable, practical lasers, and they play an important role in the field of lasers. For cw lasers, in addition to CO2 lasers, the fiber laser is the highest power laser.1–3) Since a fiber laser consists of optical fiber devices, and the output beam comes from optical fibers, it is a stable and maintenance-free laser, and the handling of the output beam is convenient. In addition, the conversion efficiency from electricity to light is high, so the fiber laser acts as a low-energy-consumption laser. These days, fiber lasers are used as the laser sources in laser processing in the field of industry.
If we set the power-dependent variable transmission element with a fast response inside the fiber laser cavity, we can achieve ultrashort pulse oscillation (passive mode-locking). Thanks to the advance of specialty fibers and related devices, the power scaling of fiber lasers has advanced step by step. Furthermore, by coupling the ultrashort pulses from fiber lasers into a variety of fibers, novel high functionality can be realized, such as ultrawideband spectral generation, wavelength-tunable ultrashort pulse generation, and pulse and spectral compression. Using these interesting light sources, we can demonstrate novel laser application technologies, especially in the field of optical metrology.
In this review paper, the development of highly functional ultrashort pulse fiber lasers and their applications are reviewed based on our achievements. First, the progress of ultrashort pulse fiber lasers is mentioned in Sect. 2. Next, the advance of fiber laser amplifiers to obtain the high power ultrashort pulse is described in Sect. 3. The extremely ultrashort pulse generation is mentioned in Sect. 4. The development of wideband fiber lasers is explained in Sect. 5. The application of highly functional lasers is briefly reviewed in Sect. 6.
2. Progress of ultrashort pulse fiber laser
In the fiber lasers, optical fibers doped with rare earth materials, such as Er and Yb, have been used as the gain devices.4) Figure 1 shows the gain spectra of representative rare-earth-doped fibers used for fiber lasers. The Er-doped fiber is the most popular fiber used as the gain device of the fiber laser. The corresponding operation wavelength range is from 1.53–1.56 µm, which is used for optical fiber communication systems. Owing to the growth of optical fiber communication technology, the related devices for Er-doped fibers have been well developed. The Yb-doped fiber is another very popular fiber used as the gain device of the fiber laser. The quantum efficiency of the Yb-doped fiber is as high as ∼80%, and the highest power of the fiber laser was achieved using the Yb-doped fiber. The Er- and Yb-co-doped fiber is also used to achieve the high gain around the 1.55 µm wavelength region.
Fig. 1. Gain spectra of representative rare-earth-doped fibers.
Download figure:
Standard image High-resolution imageBesides, Nd at a wavelength of 0.95 µm, Tm at 1.9 µm, and Pr at 1.3 µm have been developed and used as the gain fibers. Among them, the Tm-doped fiber has recently become popular for the development of the fiber laser at the 1.9 µm mid-infrared wavelength region.
In the laser cavity, when we use the element whose transmittance rapidly changes depending on the optical power, the ultrashort pulse oscillation can be achieved spontaneously. Since the phase of each oscillation mode is automatically adjusted in this process, this phenomenon is called passive mode-locking.5)
As the intensity-dependent element in the fiber laser, (1) the nonlinear effect in optical fibers, and (2) a saturable absorber are generally used.6,7) For the former, the schemes of nonlinear polarization rotation and a Fig. 8 laser using the nonlinear amplifying loop mirror are used.7) For the latter, a semiconductor saturable absorption mirror, which we call SESAM, has been mainly used. In this decade, nanographene materials, such as single-wall carbon nanotubes (SWNTs) and graphene, have attracted attention as wideband saturable absorbers. Using these intensity-dependent devices in the fiber laser cavity, and controlling the dispersion property and nonlinearity in the cavity adequately, various kinds of ultrashort pulse oscillations, such as soliton (chirp-free sech2-shaped solitary pulse), similariton (parabolic-shaped pulse with linear chirping), and dissipative soliton (linearly chirped high-energy pulse with steep edges on the pulse spectra), are obtained.
Download figure:
Standard image High-resolution imageDownload figure:
Standard image High-resolution imageFig. 8. Spectra and spectral phase of output pulses from Er-doped SWNT fiber laser when the net cavity dispersion, DT was (a) −0.032 ps2, (b) +0.017 ps2, and (c) +0.098 ps2. Figure reproduced with permission from Ref. 11. © 2011 OSA.
Download figure:
Standard image High-resolution imageIn the fiber laser, the rare-earth-doped fiber is pumped by laser diodes, and used as the gain medium. Compared with the solid-state laser using a small laser crystal, the fiber amplifier consists of a few meters long fibers. Thus, the fiber amplifier has a wide surface area, which brings us a high heat radiation efficiency. Owing to the high heat radiation efficiency, the fiber laser generally does not need water cooling, and a small and portable laser system can be demonstrated.
Figure 2 shows an example of an ultrashort-pulse Er-doped fiber laser using nonlinear polarization rotation. In this scheme, the polarization condition varies owing to the nonlinear phase shift, and the power-dependent transmission property is achieved using the combination of the polarizer, wave plates, and nonlinear polarization variation. By changing the dispersion property, we can demonstrate soliton and stretched pulse mode-locking. In Ref. 8, the average power of 100 mW and repetition rate of 50 MHz were achieved for stretched pulse mode-locking operation.
Fig. 2. Configuration of Er-doped ultrashort pulse fiber laser using nonlinear polarization rotation. HC-EDF, high-concentration Er-doped fiber, PBS, polarization beam splitter, BT, birefringent plate, WDM, wavelength division multiplexed coupler, PBC, polarization beam combiner.
Download figure:
Standard image High-resolution imageIn that scheme, the passive mode-locking is achieved using the power-dependent variation of polarization. However, environmental variation, such as temperature and humidity change, affects the birefringence of fibers and this degrades the long-term stability.
Figure 3 shows the configuration of an Er-doped ultrashort pulse fiber laser using SWNTs dispersed in polyimide film.9–11) The carbon nanotube polyimide film works as a transmission-type saturable absorber. It is easily inserted in the fiber laser cavity using the fiber connectors, as shown in Fig. 3(b). In this scheme, we can easily demonstrate the all-polarization maintaining-type fiber laser, which shows high environmental stability.
Download figure:
Standard image High-resolution imageFig. 3. (a) Configuration of Er-doped ultrashort pulse fiber laser using SWNTs dispersed in polyimide film, (b) polyimide film dispersed with SWNTs on fiber conector. NDF, normal dispersion fiber, EDF, Er-doped fiber, WDM, wavelength division multiplexed coupler.
Download figure:
Standard image High-resolution imageAnother advantage of SWNTs is their wide operation bandwidth. Figure 4 shows the characteristics of SWNT polyimide films. We used two kinds of SWNT synthesized with the high-pressure CO2 (HiPco) method and laser ablation (LA).9–11) Using the LA method, SWNTs with the diameter of 1.2 nm were selectively synthesized, and they showed an intense absorption peak at approximately 1.55 µm. Figure 4(b) shows the observed saturable absorption properties for two types of SWNT films. Compared with the SWNTs synthesized by the HiPco method, the SWNTs synthesized by the LA method show a large modulation depth of ∼20% for an irradiation power up to 5 mW. Owing to the low saturation power and large modulation depth, we can achieve passive mode-locking effectively with a low irradiation power.
Download figure:
Standard image High-resolution imageFig. 4. Characteristics of SWNT polyimide film. (a) Absorption spectra and (b) observed absorption as a function of peak power density.
Download figure:
Standard image High-resolution imageFigure 5 shows the variation of the output power and the operating mode of the SWNT Er-doped soliton mode-locked fiber laser as a function of pump power. As the pump power is increased, cw oscillation occurs, and then the operation mode is shifted to self-Q-switching, and next single pulse mode-locking is achieved. The self-start mode-locking operation is achieved stably. Above the maximum threshold of single pulse mode-locking, multiple pulse oscillation occurs.
Fig. 5. Output power and operating mode of the Er-doped SWNT soliton mode-locked fiber laser as a function of pump power. Figure reproduced with permission from Ref. 10. © 2009 OSA.
Download figure:
Standard image High-resolution imageOne of the advantages of SWNTs is their wide operation bandwidth. Figure 6 shows the variation of the optical spectra of output pulses when the center wavelength of the filter is tuned. The pulse oscillation was achieved at the whole gain bandwidth of the Er-doped fiber. Thus far, the passive mode-locking using SWNTs has been reported at a wide wavelength region from 1.06 to 1.99 µm. Now, graphene is also attracting attention as a new saturable absorber.12,13) In principle, graphene has a much wider operation bandwidth and high damage threshold compared with SWNTs. Thus, it is expected as a new saturable absorber with high potential.
Fig. 6. Variation of optical spectra of output pulses in the Er-doped SWNT soliton mode-locked fiber laser for wavelength tunable operation. Figure reproduced with permission from Ref. 10. © 2009 OSA.
Download figure:
Standard image High-resolution imageGenerally, the maximum power of an SWNT fiber laser is limited by the damage threshold of the SWNT device and the nonlinear effects inside the cavity. Thus, power scaling is one of the important points to be improved to advance fiber laser technologies.
Recently, we have investigated the dispersion management of SWNT fiber lasers and we succeeded in the large power scaling of the fiber laser.11) Figure 7 shows the variation of output properties in the dispersion managed SWNT fiber laser as a function of total dispersion DT, and Fig. 8 shows the corresponding spectra and spectral phase of output pulses. When the magnitude of total dispersion DT is negative, the soliton mode-locking occurs and almost chirp-free transform-limited sech2-shaped pulse oscillation is achieved. The ultrashort pulses were obtained in the soliton mode-locking region of DT < 0. However, the maximum pulse energy is limited by the soliton condition and the high-energy pulse cannot be achieved. For the strong positive DT region, dissipative mode-locking operation is achieved and optical pulse spectra with sharp edges and almost linear chirping are obtained. In this region, as DT was increased, the average power and the temporal width were both increased. When DT ∼ 0, it was difficult to achieve the stable single pulse mode-locking operation. From the numerical analysis, it was considered that it would be possible to achieve stable single pulse mode-locking if the modulation depth of the SWNT film was increased.
Fig. 7. Dependence of output pulses from dispersion-managed Er-doped SWNT fiber laser on the net cavity dispersion, DT. Figure reproduced with permission from Ref. 11. © 2011 OSA.
Download figure:
Standard image High-resolution imagePower scaling is an important task for fiber lasers.14,15) The obstacles were the single pulse operation limit and the damage threshold of SWNT films. We optimized the cavity configuration of the SWNT fiber laser, and recently, we have achieved the highest average power of 285 mW in dissipative soliton mode-locking operation.16) To the best of our knowledge, this is the highest power yet achieved for a fiber laser with nanocarbon materials.
As mentioned previously, the SWNT film works as a transmission-type saturable absorber, and it is useful to construct an all-polarization maintaining-type fiber laser. Using the polyimide film dispersed with SWNTs, we demonstrated an ultralow-repetition rate, high-energy, all-polarization maintaining, ultrashort pulse Er-doped fiber laser.17) A novel theta cavity configuration was proposed and a repetition rate as low as 132 kHz was demonstrated.
3. Ultrashort pulse fiber amplification
The power scaling of fiber lasers is an important subject to widen the application of fiber lasers. One of the benefits of the fiber laser is its ease of amplification by only connecting the output fiber to the input of the fiber amplifier. High-power ultrashort pulse generation using an Er-doped fiber amplifier was demonstrated by a few different groups.18–20) For the amplification of ultrashort pulses, however, nonlinear effects were induced by the amplified pulses and it is difficult to achieve high-energy ultrashort pulses. To suppress the nonlinear effects, large mode-field area fibers and rod fibers have been investigated for power scaling.3)
It is also difficult to generate clean, pedestal free, high-power ultrashort pulses in a fiber amplifier. The similariton amplifier was developed in 2000 for the first time by Fermann et al.21) The similariton amplifier generates a parabolic-shaped pulse with linear chirping property. The almost pedestal-free, ideal ultrashort pulse can be generated after the dispersion compensation. The similariton amplifier has been investigated by several groups for Yb-doped fibers in the 1.06 µm wavelength region.22,23)
There are only a few reports of a similariton amplifier for the Er-doped fiber (EDF) system.24,25) We developed a high-quality similariton amplifier based on the EDF system.26) A parabolic-shaped, high power, almost ideal similariton pulse was generated in EDF. After the dispersion compensation, a 4.4 nJ, 51 fs, almost pedestal-free, high-power, ultrashort pulse was generated.
The experimental setup is shown in Fig. 9. The output pulse from a passively mode-locked Er-doped ultrashort pulse fiber laser was coupled into a positively dispersive Er-doped fiber through a WDM coupler. In the EDF, the sech2 pulse is amplified and transformed into parabolically-shaped similariton pulses.
Fig. 9. Configuration of similariton amplifier and SC generation. PBC, polarization beam combiner, PC, polarization controller, WDM, wavelength division multiplex coupler, EDF, Er-doped fiber, LMA-PCF, large mode area photonic crystal fiber, HNLF, highly nonlinear fiber. Cited from Ref. 26. © The Japan Society of Applied Physics.
Download figure:
Standard image High-resolution imageFigure 10 shows the variation of the optical spectra of the output pulses from the EDF for different pump powers. The input power was 2 mW. As the pump power was increased, the spectra were broadened monotonically, and almost parabolically-broadened pulse spectra were generated. When the pump power was maximum, the output power was 235 mW and the pulse energy was 4.9 nJ. A spectral width of 90 nm was achieved.
Fig. 10. Optical spectra of output of similariton amplifier for each pump power condition. Cited from Ref. 26. © The Japan Society of Applied Physics.
Download figure:
Standard image High-resolution imageFigure 11 shows the temporal shape and phase of the dispersion-compensated pulse using LMA-PCF. An almost pedestal-free ultrashort pulse was generated. The temporal width was 51 fs and the peak power was up to 80 kW. The pulse energy was 4.4 nJ. Although a small pedestal component ∼2% remained, almost an ideal ultrashort pulse was generated in the Er-doped similariton amplifier. Using the generated high-energy ultrashort pulse, an octave spanning coherent supercontinuum was successfully generated, as mentioned later.
Fig. 11. Temporal shape of dispersion compensated pulse from similariton amplifier. Cited from Ref. 26. © The Japan Society of Applied Physics.
Download figure:
Standard image High-resolution image4. Extremely ultrashort pulse generation
The realization of a practical extremely ultrashort pulse source is desired in the fields of ultrashort pulse laser applications. Figure 12 shows the experimental setup of 13 fs extremely short pulse generation using an Er-doped fiber laser and hybrid fibers.27) The output pulse with a temporal width of 100 fs from the ultrashort pulse Er-doped fiber laser was coupled into hybrid fibers, which consist of a polarization maintaining fiber and a highly nonlinear fiber. The length of each part was designed using numerical simulation.
Fig. 12. Experimental setup of 13 fs ultrashort pulse generation using hybrid fibers. PMF, polarization maintaining fiber, HNL-DSF, highly nonlinear dispersion shifted fiber.
Download figure:
Standard image High-resolution imageAt the initial PMF, the pulse compression occurs through higher-order soliton compression by the combination of anomalous dispersion and self-phase modulation. The temporal width was compressed from 107 into 34 fs. Then, the compressed pulse was introduced into the highly nonlinear dispersion shifted fiber, and the optical spectrum was broadened through self-phase modulation. Finally, the chirping was compensated using a collimated lens, and an extremely short pulse with a temporal width of 13 fs was generated, as shown in Fig. 13. The temporal width of the corresponding transform-limited pulse was 11 fs, and we can see that the generated 13 fs pulse was close to the transform limited pulse.
Fig. 13. Temporal pulse shape and phase of 13 fs extremely short pulse generated with Er-doped ultrashort pulse fiber laser and hybrid fibers. The inset shows the corresponding transform limited pulse.
Download figure:
Standard image High-resolution imageIn the all-fiber systems, it is difficult to generate pedestal-free extremely short pulses. One of the reasons for this is the pulse distortion owing to the induced nonlinear effects in the fibers. Another is the difficulty in the compensation of higher-order dispersion in the fibers.
In 2006, we succeeded in the generation of a pedestal-free 22 fs ultrashort pulse in all-fiber systems.28) The experimental setup is shown in Fig. 14. The 260 fs ultrashort pulse from a passively mode-locked Er-doped fiber laser with a repetition rate of 48 MHz was introduced into the chirped pulse fiber amplifier system, and a high-power Raman shifted soliton pulse was generated. A 110 fs, 60 mW, clean sech2-shaped pulse was generated at the wavelength of 1690 nm. Then, the generated soliton pulse was coupled into hybrid fibers to generate extremely short pulses. First, the soliton pulse was introduced into a normal dispersion highly nonlinear fiber, and the pulse spectrum was broadened.
Fig. 14. Setup of 22 fs pedestal-free ultrashort pulse in Er-doped fiber system. (a) High-power Raman soliton pulse generation stage, and (b) pulse compression stage. SMF, single mode fiber, EDFA, Er-doped fiber amplifier, WDM, wavelength division multiplexed coupler, ND-HNLF, normal dispersion highly nonlinear fiber, RDF, reverse dispersion fiber.
Download figure:
Standard image High-resolution imageThen, the generated spectrally broadened pulse was introduced into the 2nd stage of the reverse dispersion fiber (RDF). The SMF and ND-HNLF have a positive dispersion slope. On the other hand, the RDF has anomalous dispersion and a negative dispersion slope. So, using RDF, we can compensate the mismatch of the dispersion slope, which corresponds to the compensation of the 3rd-order dispersion. After RDF, the optical pulse was introduced into SMF, and the extremely short pulse was obtained.
Figure 15 shows the temporal and spectral shapes of the generated ultrashort pulse observed with SHG-FROG. Owing to the ideal spectral shape and accurate dispersion compensation, the almost chirp- and pedestal-free 22 fs ultrashort pulse was obtained. From the numerical analysis, it was predicted that if the RDF was not used, a wider pulse with a temporal width of 30 fs and a moderate pedestal component would be generated. A similar ultrashort pulse generation system was applied as the light source for wideband THz generation.29,30)
Fig. 15. (a) Observed temporal shape of dispersion-compensated ultrashort pulse, and (b) pulse spectrum and delay time for generated 22 fs pedestal-free ultrashort pulse. The pulse was observed using SHG-FROG and reconstructed with FROG algorism.
Download figure:
Standard image High-resolution imageThe challenge to generate the shortest pulse has been investigated continuously. Recently, extremely short pulse generation has been reported using two color SCs.31) The two color SCs were generated using the carrier envelope offset frequency (fceo) locked fiber laser system, and then they were temporally overlapped. Although lots of bulk optics remained, a 4.3 fs extremely short pulse was generated in the fiber-laser-based system. A more practical system is desired in terms of the ultrashort pulse applications.
5. Wideband fiber lasers
5.1. Wavelength tunable ultrashort pulse fiber laser
As mentioned before, optical fibers are ideal, ultra-low-loss waveguides, and we can confine the light beam inside the small core area along the long propagation length. So, although the nonlinear refractive index itself is not so large, optical fibers work as one of the most effective nonlinear optical devices. We have been investigating widely wavelength tunable ultrashort pulse sources.32)
Figure 16 shows the variation of the optical spectra of a wavelength tunable soliton pulse source.33–35) When the intense pulse propagates along the anomalous dispersion fiber, the soliton effect, which is the combination of self-phase modulation and anomalous dispersion, is induced and pulse compression occurs. During the pulse compression, the spectrum is broadened by self-phase modulation. In the broadened spectrum, stimulated Raman scattering is induced and the longer wavelength side suffers the Raman gain of the shorter wavelength component. As a result, the shorter wavelength components are transferred into the longer wavelength ones, and pulse breakup occurs and a new ultrashort pulse is generated at the longer wavelength side. This pulse suffers the soliton effect and a sech2-shaped ultrashort pulse is generated. Inside this pulse, stimulated Raman scattering is also induced and the center wavelength of the soliton pulse is continuously shifted towards the longer wavelength side during the propagation along the fiber. The magnitude of the wavelength shift depends on the fiber input power and fiber length. Using the power control, we can demonstrate rapid, wideband wavelength tunable operation.36,37)
Fig. 16. Variation of optical spectra of wavelength-tunable soliton pulse source. The corresponding fiber input power is shown above each spectrum.
Download figure:
Standard image High-resolution imageThe phenomenon of soliton self-frequency shift was discovered in 1986 by Mitschke and Mollenauer.38) In the initial stage, the amount of wavelength shift was less than 50 nm. In 1999, Nishizawa and Goto demonstrated the wideband wavelength tunable pulse source using an ultrashort pulse fiber laser and a small core PM fiber, and it attracted a lot of attention.33–35) Using an Yb-doped fiber laser and photonic crystal fibers, we achieved the wide wavelength tuning from 1.06 to 1.9 µm.37,39)
Using the birefringent fibers, we can generate two color soliton pulses simultaneously.40) In the birefringent fiber, the orthogonally polarized pulses propagate independently, and each pulse generates a Raman shifted soliton pulse. The wavelength of the two pulses can be tuned by varying the fiber input power and polarization direction of the input beam, as shown in Fig. 17.
Fig. 17. Simultaneous two color soliton pulse generation using birefringent fiber. The magnitude of θ represents the polarization angle of the input pulse with respect to the birefringent axis of the fiber.
Download figure:
Standard image High-resolution imageAs mentioned above, we can generate a continuously red-shifted ultrashort soliton pulse using the soliton self-frequency shift. In addition, we can demonstrate the continuous blue-shift of the optical pulse using the interaction of the soliton pulse. Figure 18 shows the variation of the optical spectra. An ultrashort soliton pulse in the anomalous dispersion region and a short pulse in the normal dispersion region are coupled into the fibers. When the temporal relation between the two pulses is adjusted and the soliton pulse and the short pulse are temporally overlapped, if the group velocity matching condition is satisfied between the two pulses, the soliton pulse traps the other pulse through the cross-phase modulation, and they copropagate along the fibers. As the power of the soliton pulse is increased and its wavelength is red-shifted, the wavelength of the trapped pulse is blue-shifted to satisfy the group velocity matching condition. This pulse trapping phenomenon was discovered in 2002 by Nishizawa and Goto.41,42) Using this phenomenon, the wavelength tuning at 1.32–1.5 µm, and 0.45–0.53 µm was demonstrated.43,44)
Fig. 18. Variation of optical spectra for pulse trapping, (a) fiber input, and (b, c) output spectra when input power of soliton pulse was (b) 2 mW and (c) 4 mW. The broken line shows the group delay of the corresponding fibers.
Download figure:
Standard image High-resolution imageThe wideband wavelength tunable source is attractive for spectroscopic applications. For the spectroscopic applications using a wavelength tunable light source, the spectral resolution is determined by the instantaneous spectral width of the light source, and a narrower spectral width is preferred for high-resolution measurement. Recently, we have demonstrated the higher-order spectral compression using the comb-profile fiber technique.45) Figure 19(a) shows the dispersion profile of the designed comb-profile fiber. We designed the quasi dispersion increasing fiber using the combination of a conventional single mode fiber and a dispersion shifted fiber using the numerical simulation. The adiabatic soliton spectral compression occurs along the fiber, and an ideal narrow band spectrum was obtained. Figure 19(b) shows the numerical results of spectrum compression in the designed comb-profile fiber. Thanks to the soliton effect, narrow linewidth spectra with small pedestal components were obtained. The numerical results were well in agreement with the experimental ones.
Download figure:
Standard image High-resolution imageFig. 19. (a) Profile of actual and averaged second-order dispersion of comb-profile fiber at each segment, and (b) numerical results of spectral compression in comb-profile fiber. Blue and red lines correspond to the input and output pulses, respectively.
Download figure:
Standard image High-resolution imageThe comb-profile fiber works for wide wavelength regions. Figure 20 shows the experimental result of the variation of the optical spectra for a wideband wavelength tunable narrow bandwidth source. The wavelength tunable soliton pulse was coupled into the comb-profile fiber and spectrum compression was induced. We achieved wideband operation at 1620–1850 nm. The largest spectrum compression ratio was 25.9. Using the developed widely wavelength tunable narrow linewidth source, ultrafast, real-time absorption spectroscopy was demonstrated.46)
Fig. 20. Variation of optical spectra of wavelength-tunable narrow-linewidth source generated with wavelength tunable soliton pulse and comb-profile fiber.
Download figure:
Standard image High-resolution image5.2. Wideband supercontinuum generation
Supercontinuum (SC) is the widely broadened spectrum generated by strong nonlinear effects. It is a novel light source, which works as an ultrawideband laser source. As the wideband light source, lamp sources have been mainly used. However, the emission power is low and the emission area is wide, and it is difficult to focus the beams. The SC is output from the small core of the fibers, and the output power is high, so that the SC is a useful light source for practical applications.
The first demonstration of SC was reported by Alfano and Shapiro in 1969.47) At that time, they needed mJ pulses to generate SC. Then, Mori et al. in NTT generated an SC that covered the 1.5–1.6 µm wavelength region for optical communication.48) In 2000, Ranka et al. succeeded in the generation of 0.4–1.4 µm ultrawideband SC using PCF and Ti:Sapphire lasers.49) Then, Birks et al. also demonstrated similar ultrawideband SC generation using tapered fibers.50) In these works, only sub-nJ pulses were used to generate ultrawideband SC. In 2001, Nishizawa and Goto demonstrated the 1.0–2.0 µm ultrawideband SC generation using highly nonlinear fibers and compact ultrashort pulse fiber lasers.51)
Figure 21 shows the variation of the optical spectra for ultrawideband SC generation using highly nonlinear fibers and an ultrashort pulse fiber laser.51) The highly nonlinear fiber has the conventional structure, but the core diameter is small and the nonlinear refractive index is increased by the control of doping materials. The zero-dispersion wavelength is shifted to the 1.55 µm wavelength region. When the ultrashort pulse is coupled into the highly nonlinear fibers, the optical spectrum is initially broadened by self-phase modulation. Regarding the spectrum shape, several corresponding peaks are observed. As the fiber length is increased, four-wave mixing (FWM) and stimulated Raman scattering become effective, and the bandwidth is broadened and the spectrum shape is gradually flattened. When the fiber length was 5 m, a widely broadened SC with good flatness was generated. The average power was 30 mW.
Fig. 21. Ultrawideband supercontinuum generation in highly nonlinear dispersion shifted fiber for the fiber lengths of 1, 2, 3, and 5 m. Cited from Ref. 51. © The Japan Society of Applied Physics.
Download figure:
Standard image High-resolution imageFigure 22 shows the optical spectrum of a 0.45 to 1.4 µm widely broadened SC based on the Er-doped fiber laser.52) The output of the Er-doped fiber laser is amplified by the Er-doped fiber amplifier, and then it was focused into a periodically poled LiNbO3 (PPLN) and an SHG pulse was generated. Then, the generated SHG pulse was coupled into the PCF and a widely broadened SC was generated. The wavelength range was from 0.4 to 1.4 µm, and a widely broadened SC covering the visible to NIR range was generated. Thanks to the development of the PCF and highly nonlinear fibers, and the advance of the ultrashort pulse fiber laser, we can generate a widely broadened SC with a pulse energy on the order of nJ, which is about 1/100 compared with the situation a decade ago.
Fig. 22. 0.45–1.40 µm visible — near infrared ultrawideband SC generated with ultrashort pulse fiber laser system. Broken line shows the spectrum of SHG pulse used for pumping of PCF.
Download figure:
Standard image High-resolution imageThe SCs shown in Figs. 21 and 22 look good, but strictly speaking, they have inherent large noise and low coherence. It was also clarified that there exist lots of unstable fine structures on the spectrum shape.
A few groups including ours investigated the SC generation process.53,54) Since the optical fiber is an ideal waveguide, the numerical analysis is a useful tool to investigate the generation process and physical mechanism of SC.55) Figure 23 shows a numerical result of the temporal distribution of the spectrum component, which we call a "spectrogram", for SC generated in 10 m of a highly nonlinear dispersion shifted fiber. The horizontal and vertical axes represent the time and the frequency (wavelength), respectively. We can see that the spectrum components are broadened parabolically and interactions occur between the temporally overlapped components. As a result, fine components are generated on the spectra and they increase the noise and degrade the coherence properties.
Fig. 23. Numerical result of SC generated in 1 m of highly nonlinear dispersion-shifted fiber. Spectrogram (temporal distribution of spectral components) and temporal and spectral properties are represented. Soliton pulses and trapped pulses are generated at longer and shorter wavelength sides, respectively.
Download figure:
Standard image High-resolution imageIt is interesting to note that the soliton pulse at the longer wavelength side traps the temporally overlapped shorter wavelength components and their wavelength is blue-shifted.41) This is the mechanism of the spectrum broadening of SC at the shorter wavelength side.54)
We have tackled the problem of noise increment and the degradation of coherence,56) and we have succeeded in the development of a fiber-laser-based high-quality SC source.57) The output pulses from the Er-doped fiber laser were amplified and then Raman shifted soliton pulses were generated in the following single mode fibers. Raman shifted soliton pulses have an ideal chirp-free sech2-shape without pedestal, and work as ideal pump pulses for SC generation. Then, the generated pulses were coupled into a normal dispersion highly nonlinear fiber, and a flatly broadened SC was generated. In this scheme, the monotonic chirping property is achieved, and the noise increment can be avoided. Figure 24 shows the optical spectra of the generated high-quality SC. The spectral shapes are shown on linear and log scales. The FWHM bandwidth was 580 nm and the spectral flatness was ±1 dB. We also examined the noise and coherence properties, and we confirmed that the generated SC was a low-noise, highly coherent, high-quality SC.
Fig. 24. Observed spectra of high quality coherent SC shown on linear and log scales. Cited from Ref. 57. © The Japan Society of Applied Physics.
Download figure:
Standard image High-resolution imageAn octave spanning coherent SC is important for the frequency stabilization of the optical frequency comb system. We generated an octave spanning high-quality SC using a hybrid configuration of highly nonlinear fibers.58) Recently, we have succeeded in the generation of high-energy, clean ultrashort pulses in a similariton amplifier, as mentioned in Sect. 3.26) Figure 25 shows the optical spectra of the SC generated with a normal dispersion highly nonlinear fiber and a high-energy ultrashort pulse from a similariton amplifier. A smoothly broadened, coherent, low noise SC was generated through the combination of self-phase modulation and normal dispersion. An octave spanning coherent SC broadened from 1.05 to 2.15 µm was successfully generated using only the normal dispersion highly nonlinear fiber.
Fig. 25. Optical spectra of generated octave spanning coherent SC generated in normal dispersion highly nonlinear fiber. Cited from Ref. 26. © The Japan Society of Applied Physics.
Download figure:
Standard image High-resolution image6. Applications
Fiber lasers are the most practical high-power lasers and are now used as laser sources in a variety of laser applications. One of the most popular applications is laser processing. At present, fiber lasers are the highest power lasers in addition to the CO2 laser.1) As one of the advantages of fiber lasers, the output beam is irradiated stably from the small circular core. So, the output beam can be focused on a small spot stably. The high efficiency of fiber lasers is also a good benefit for energy saving. Since there is almost no bulk component, fiber lasers show excellent environmental stability and they work as maintenance-free lasers. Now, the number of fiber lasers used in laser processing as practical laser sources is increasing. Using the ultrashort pulse, a precise process without a thermal effect can be demonstrated.
Fiber lasers are used for many kinds of laser applications. One of the fields is "imaging". Ultrashort pulse fiber lasers have been used for several kinds of nonlinear laser microscopies.59,60) Fiber lasers are useful as practical and functional laser light sources.
We have been investigating ultrahigh resolution (UHR) optical coherence tomography (OCT) using a fiber SC.8,61–63) The theoretical axial resolution of OCT is determined by the spectral width of the light source. Using the ultrashort pulse fiber laser and nonlinear fiber technologies, we can demonstrate practical, fiber-based, high-power, wideband sources.
One of the important tasks of OCT is the increment of penetration depth. The penetration depth is mainly limited by the absorption of water and hemoglobin, and scattering in the tissue. The magnitude of scattering depends on the wavelength, and it is decreased as the wavelength is increased. In terms of the scattering, a larger penetration depth may be achieved in a longer wavelength region.
We have been investigating UHR-OCT using fiber lasers. We demonstrated UHR-OCT in the 1.7 µm wavelength region for the first time.63) Then, to discuss the wavelength dependence of OCT imaging, we developed SC sources and corresponding OCT systems in five different wavelength regions from the 800–1700 nm region.64)
Figure 26 shows an example of UHR-OCT images observed with the fiber laser SC at 1.7 µm wavelength. A high-power, low-noise, Gaussian-like SC was generated using an SWNT fiber laser and an Er-doped fiber amplifier.65) The SC source consists of a shoe-box-sized fiber laser and an SC generation system, so it is portable. For the OCT imaging, the axial resolution was 3.2 µm in tissue and the sensitivity was 105 dB. The precise structures of human nail and pig thyroid gland were clearly observed. We confirmed that the higher penetration depth was achieved in a longer wavelength system for low-water-absorption samples.
Download figure:
Standard image High-resolution imageFig. 26. UHR-OCT images of (a) human nail and (b) pig thyroid gland observed with fiber laser SC at 1.7 µm wavelength. Important structures inside the sample were observed. Er, epidermis, D, dermis, Np, nail plate, Nb, nail bed.
Download figure:
Standard image High-resolution imageUltrashort pulse fiber lasers are also useful as a practical optical frequency comb system. The optical frequency comb works as the optical frequency standard, and it enables us to demonstrate precise spectroscopy and highly accurate distance measurement. Figure 27 shows an example of the observed fceo beat signal in the optical frequency comb system based on an ultrashort pulse fiber laser. The ultrashort pulse fiber laser works as a stable and practical optical frequency comb system. The international standard of length is now determined by the fiber laser optical frequency comb system.66)
Fig. 27. RF beat spectra of carrier envelope offset frequency (fceo) in fiber-laser-based optical frequency comb system.
Download figure:
Standard image High-resolution imageThe ultrashort pulse fiber laser is also used as the pulse source for THz generation and detection.29,30) The fiber laser now works as a practical laser, and it has advanced the field of laser applications.
7. Conclusions
Fiber lasers, which consist of ultra-low-loss optical fibers, work as stable, compact, and practical lasers. Nowadays, fiber lasers are the most practical lasers in many fields. In this paper, the progress of highly functional ultrashort pulse fiber laser sources and their applications were reviewed on the basis of the works of this author.
In Sect. 2, the progress of ultrashort pulse fiber lasers was reviewed. Recently, a compact, stable, ultrashort pulse fiber laser has been demonstrated using carbon nanotubes dispersed in polyimide film. The highest power operation of a nanocarbon fiber laser was demonstrated by the optimization of fiber laser cavities.
In Sect. 3, the advance of ultrashort pulse amplification techniques was discussed. A pedestal-free, 4.4 nJ, 51 fs ultrashort pulse was generated based on a similariton amplifier. An octave spanning coherent supercontinuum was generated using the developed pulse source and highly nonlinear fibers.
In Sect. 4, the extremely ultrashort pulse generation based on an ultrashort pulse fiber laser system was mentioned. A 13 fs extremely short pulse, and a 22 fs pedestal-free high-energy pulse were successfully generated based on the all-fiber systems at around the 1.55 µm wavelength region.
In Sect. 5, the progress of wideband fiber lasers was reviewed. In Sect. 5.1, widely wavelength tunable ultrashort pulse generation was discussed. Red-shift, blue-shift, and two color generation were demonstrated. Recently, a novel spectral compression technique has been developed using the comb profiled fiber technique. An ultrafast, wideband wavelength tunable, narrow linewidth source was successfully demonstrated.
In Sect. 5.2, the advance of widely broadened supercontinuum generation was described. Since 2000, ultrawideband supercontinuum generation has been realized owing to the advance of ultrashort pulse lasers and highly nonlinear fibers. As the results of investigations, we succeeded in coherent, low noise, almost flat, ideal supercontinuum generation using the combination of a pulse shaping technique and the usage of adequate highly nonlinear fibers.
In Sect. 6, the applications of ultrashort pulse fiber lasers were reviewed. Fiber lasers are one of the highest power lasers, and they have been used as the light source in laser processing. The highly functional fiber lasers are useful for imaging and metrology, such as optical coherence tomography (OCT), nonlinear microscopy, and optical frequency combs. The ultrahigh-resolution OCT using a fiber supercontinuum was demonstrated at several different wavelength ranges by the authors.
Fiber laser sources are practical and promising laser sources, and they also induce the advance of the fields of laser applications. Highly functional fiber lasers have excellent high potentials, and the innovation of novel technologies is expected.
Acknowledgments
The author would like to thank T. Goto in Chubu University, J. G. Fujimoto in MIT, and K. Itoh in Osaka University for their encouraging discussions. The author would like to thank Y. Sakakibara, E. Omoda, and H. Kataura for their collaboration on SWNT fiber lasers. The author also thanks all his coworkers and students for their cooperation.
Biographies
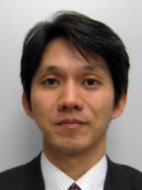
Norihiko Nishizawa is a professor in the Department of Quantum Engineering, Graduate School of Engineering, Nagoya University, Japan. His current research interests include highly functional ultrashort pulse fiber lasers and applications for imaging, especially ultrahigh resolution optical coherence tomography. He is also interested in ultrafast nonlinear optics, and highly functional optical control.