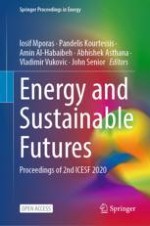
Open Access 2021 | OriginalPaper | Chapter
12. GB Grid 9 August 2019 Power Outage and Grid Inertia
Author : Christian Cooke
Published in: Energy and Sustainable Futures
Publisher: Springer International Publishing
Activate our intelligent search to find suitable subject content or patents.
Select sections of text to find matching patents with Artificial Intelligence. powered by
Select sections of text to find additional relevant content using AI-assisted search. powered by